Seven Wonders of Biology
A decades-old essay offers newfound appreciation for progress.
In 1983, a New York physician named Lewis Thomas, moonlighting as an essayist for The New England Journal of Medicine, published a collection entitled Late Night Thoughts on Listening to Mahler’s Ninth Symphony. One of its gems, “Seven Wonders,” has long lingered in my mind.
The essay’s premise is simple. Thomas has received an invitation from a magazine editor to help replace the ancient Seven Wonders with seven modern ones. Unable to settle on a list of man-made monuments, however, Thomas chooses instead to make a list of natural Wonders. His final list features everything from extremophiles thriving in boiling water to an olfactory receptor cell capable of summoning vivid memories with a single whiff.
Four decades have passed since Thomas’ essay was first published, and the pace of biological discovery has only accelerated. And so I can’t help but wonder: Which parts of biology do I consider most striking today, and how far has the field come since 1983?
Thomas skipped over the first wonder in his original list, and began instead with the second: microbes that flourish in “superheated seawater” spewed from deep sea vents at temperatures well above 300°C. Proteins and DNA usually unravel in such scalding environments, yet researchers who descended in submarines in 1982 found entire colonies thriving at these extreme temperatures.
These so-called “extremophiles” can survive extreme heat, we now know, because they carry enzymes braced by extra salt bridges, disulfide bonds, and tightly packed hydrophobic cores. They also shield their genomes using an enzyme called reverse gyrase, which burns ATP energy molecules to wrap DNA in a heat-protective supercoil.1
Like Thomas, I marvel at the diversity of lifeforms on Earth. But even more striking, for me, is the fact that so many tools used in biotechnology today came from peculiar organisms that exist on the periphery of scientific investigation. CRISPR systems drew the attention of scientists in 2007, for example, when workers studying yogurt-producing bacteria at DANISCO noticed that their microbes were resistant to bacteriophage infections. Green fluorescent protein came from a bioluminescent jellyfish captured off the coast of Washington state. Time and time again, nature rewards those with the courage to look more closely; especially in places where others have not.
Thomas’ Third Wonder was a peculiar species of beetle that climbs mimosa trees, lays its eggs, and chews on the bark to give its larvae a head-start for feeding on dying wood. All of these actions—from the specific choice of tree to the purposeful way it chews the bark—are “hardwired” into the beetle’s DNA. But “what are the odds favoring three totally separate bits of behavior,” Thomas muses, “happening together by random chance among a beetle's genes?”
I don’t know nearly enough about evolutionary biology to answer this question. But what I do know is that one does not need to study beetles to marvel at the complexity of evolutionary outcomes; a single cell will suffice.
I find it wondrous that every living cell on Earth emerges from a “bag” of jostling molecules. Life is not “machine-like,” but amorphous and stochastic. Everything in a cell can be reduced to chemical equations.2 And yet, despite this illusion of simplicity, even the simplest of organisms—like E. coli—is capable of purposeful intelligence. Even a feat as straightforward as cells swimming toward food and away from dangerous chemicals is “intelligence in one of its most elemental forms,” according to writers James Somers and Edwin Morris.
“An individual E. coli has no brain,” the duo writes, “and is even many orders of magnitude simpler than a human cell, and yet already it possesses something like a sense of smell, drive, even a memory.” When E. coli senses it’s getting close to a food source, it swims in a straight line. When it senses it’s moving further away, chemoreceptor proteins activate a protein called CheA, which tags another protein, CheY, to induce tumbling. The cell then proceeds to swim in a new direction. An E. coli thus hunts for its food using little more than the movements of atoms between proteins. Marvelous.
Thomas’ Fourth Wonder was a prion, a protein that can flip into a self-propagating shape. In its abnormal form, prions collide with other copies of the same protein, causing them to misfold into the same abnormal template. Skeptics ridiculed Stanley B. Prusiner, a researcher at the University of California, San Francisco, when he first proposed “proteinaceous infectious particles.” To those skeptics, it seemed ludicrous that a single protein could be infectious in the absence of DNA or RNA. But Prusiner persisted, eventually earning a Nobel Prize for revealing how prions cause Creutzfeldt-Jakob and Mad Cow disease.
Prions challenged the status quo, overturning much of what biologists thought was possible. In that sense, prions are similar to cable bacteria, an organism that forces researchers to reconsider how microbes work together in elaborate communities.
Researchers in Aarhus, Denmark first encountered cable bacteria in lake sediments. The filamentous microbes—which resemble human hair—link together in centimeters-long chains, comprising thousands of cells stacked end to end.
In these chains, cells near the middle form physical structures that allow electrons to flow through the length of the filament. These structures are built from protein-based fibers running through the periplasm (the space between the inner and outer membranes) and act like little “conduction channels.”
One tip of the microbial chain oxidizes (or strips electrons from) sulfide in the mud. These electrons then race up the chain, toward the surface, where the cell on the other tip reduces oxygen or nitrate. By creating this electron bridge, cable bacteria have evolved a way to spatially separate redox reactions to power their collective metabolism. This stands in stark contrast to a long-held assumption in biology that every living cell independently generates its own energy supply.
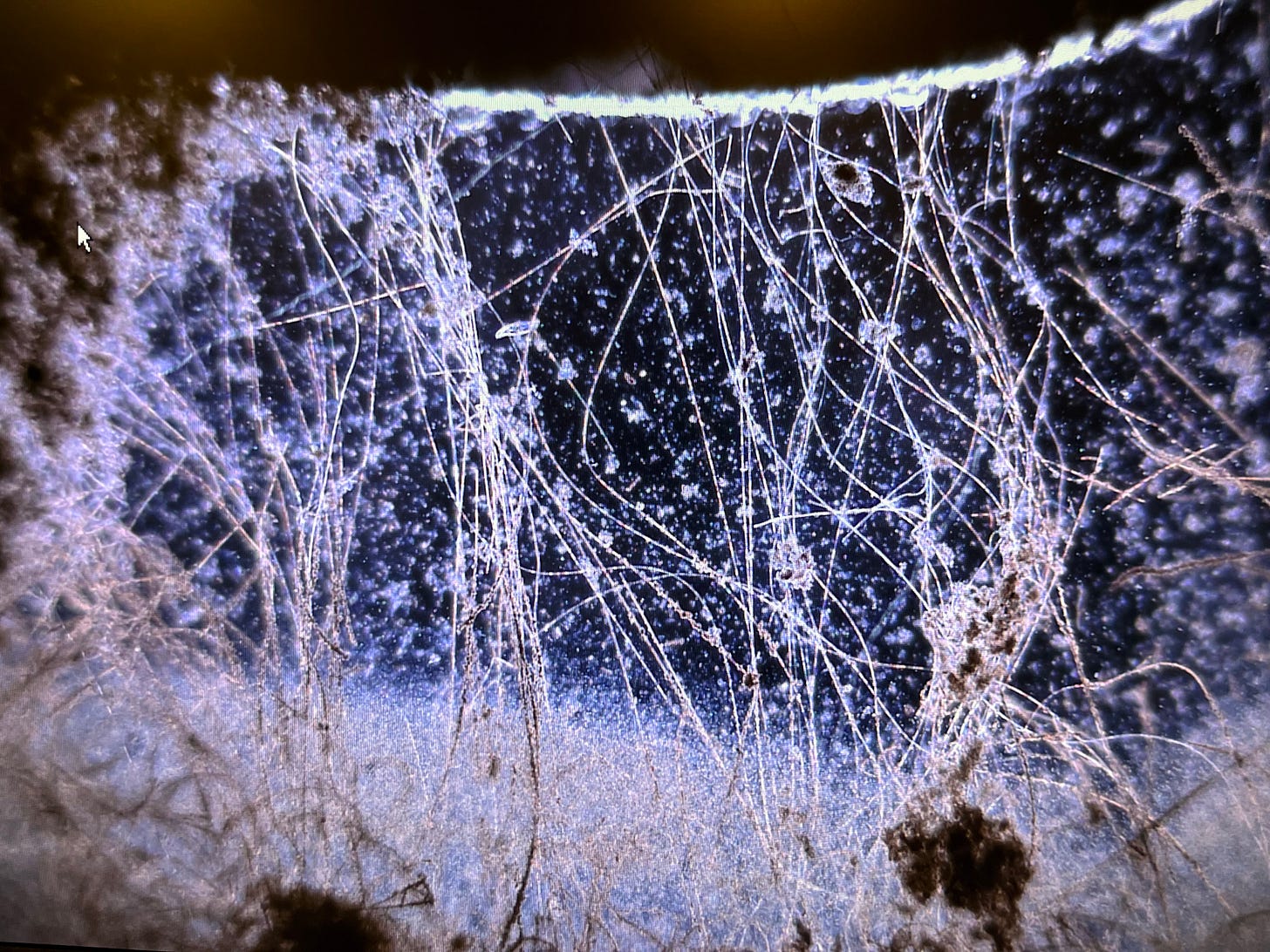
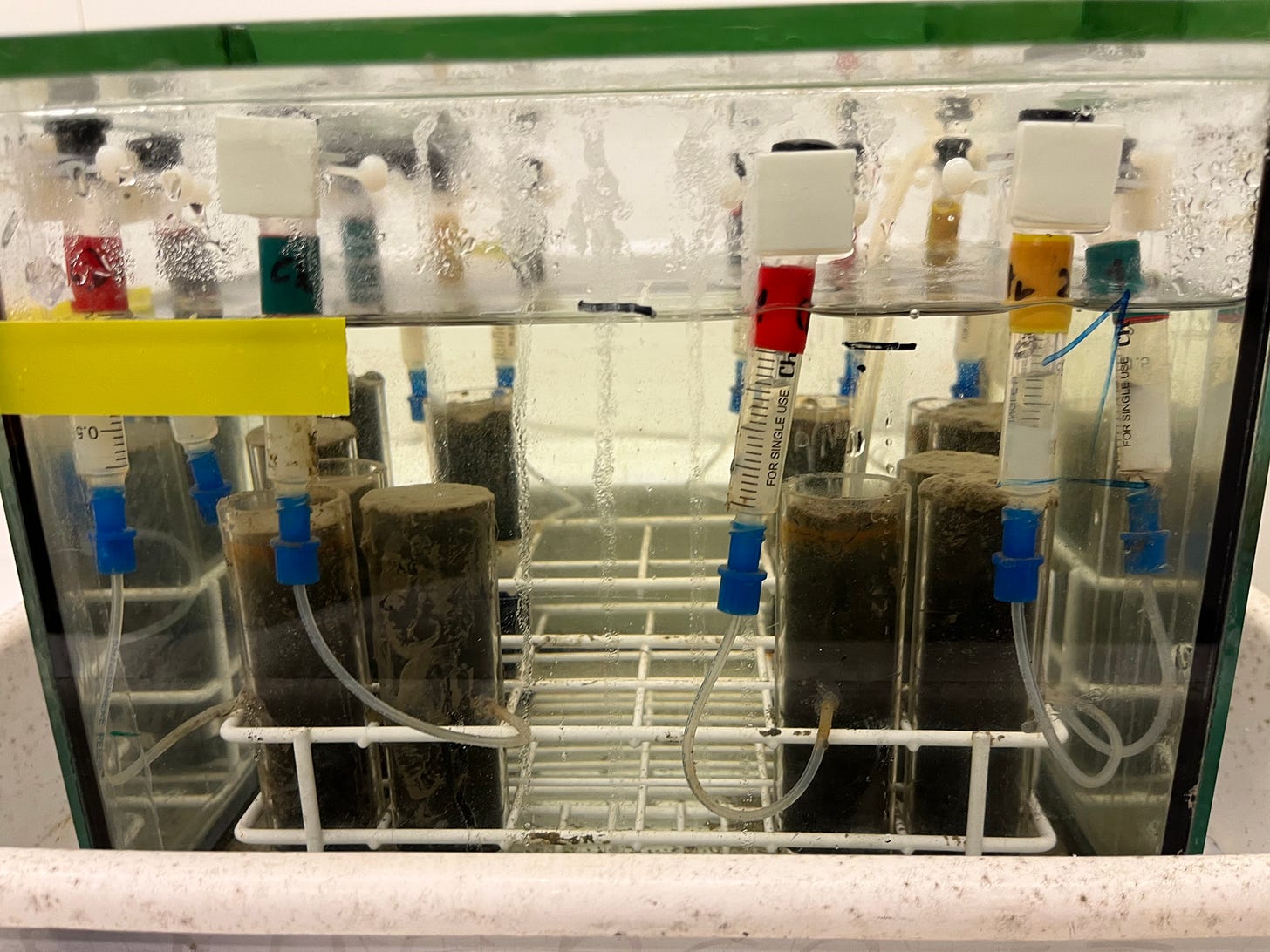
Thomas’ Fifth Wonder was an olfactory neuron. He envisioned a single nerve cell out in the open air, sampling scents and triggering memories lodged deep in the brain. In the 1970s, neuroscientists could only guess at how odors became neural signals. But today, we know that specialized receptors bind particular molecules and then spark electrical impulses. And we can even manipulate those neurons directly using optogenetics, by shining light on genetically modified cells and coaxing them to fire.
Whereas Thomas marveled at the complexity of a single neuron, I marvel at how our understanding of neurobiology has rapidly expanded to entire connectomes, or “wiring diagrams” of the brain. In the 1970s, Sydney Brenner painstakingly sliced Caenorhabditis elegans worms into micro-thin sections and photographed each one, finally revealing the animal’s 302 neurons and 7,000 connections by 1986.
Fast forward to 2024, and researchers have published a “neuron-by-neuron and synapse-by-synapse roadmap” of an entire adult fruit fly brain. The atlas spans 140,000 neurons and 50 million synapses. One can even wander through this map virtually, and test how stimulating certain neurons might affect a digital fly’s behavior. Researchers now dream of mapping the human connectome’s 100 billion neurons—a Sisyphean task. Yet if they succeed, we may someday preserve human thoughts on hard drives or figure out how neural circuits kindle a memory.
Thomas’ Sixth Wonder? Termites. “Not the single insect,” but rather “the collectivity.” He marvels that hordes of blind individuals methodically raise towering mounds, forging interconnected tunnels that automatically regulate both temperature and humidity. I find a similar fascination in rove beetles, one of Earth’s largest animal families with at least 66,000 known species (or about one-fourth of all known lifeforms on this planet).
Some rove beetles infiltrate ant colonies by synthesizing deceptive compounds that match the ants’ nestmate recognition cues. But in Southern California, a rove beetle species called Sceptobius lativentris uses a different strategy entirely. Instead of producing its own chemicals, it clambers onto the bodies of ants and repeatedly “grooms” them, transferring the ants’ pheromones onto itself. The beetle then waltzes into the nest and feasts on the ants’ eggs, or even follows ant pheromone trails to infiltrate nearby colonies. Using a molecular key, rove beetles can thus infiltrate entire ant colonies. I marvel at such complex forms of chemical communication, where the same evolutionary outcome that made ants a formidable collective can also be hijacked by invaders.
Thomas’ Seventh Wonder is humanity (quite broad, I know). He celebrates our astonishing capacity to create and reflect, but also criticizes our reckless expansion and endangering of ecosystems. I wonder, similarly, what will happen as science expands into new unknowns.
In just a few years, scientists seem likely to crack in vitro oogenesis, a technology to turn stem cells into viable eggs. This technology would expand the time frame during which women can have children or allow same-sex couples to have biological children. While I marvel at human ingenuity, and our ability to replicate what nature has solved over billions of years of evolution, it also raises difficult ethical questions: could assembly-line egg production commodify human evolution? What happens when eggs become so simple to create that any parent can afford to select those with their favorite qualities? I fear a future wherein “randomness” is removed from the human story; where embryos are edited not to prevent disease, but to design physical traits.
And so we arrive at the Number One Wonder. For Thomas, it is the fact that all life on Earth is interconnected. The entire planet, writes Thomas, “is a living system, an immense organism, still developing, regulating itself, making its own oxygen, maintaining its own temperature, keeping all its infinite living parts connected and interdependent, including us. It is the strangest of all places, and there is everything in the world to learn about it.”
It is the last bit of this sentence that I marvel at most. The job of a scientist is to keep peeling back layers in order to further our understanding, and yet it seems biology’s layers persist forever. Every time we declare ourselves “done,” we discover yet more nuance. Biologists once thought they had a comprehensive “gene list” in the wake of the Human Genome Project, only to find reams of regulatory RNAs, subtle epigenetic markers, and entire classes of small proteins they had previously overlooked.
What fascinates me just as much as how we keep stumbling upon what was always there, however, is the way that science itself morphs over time. There is a “slope toward elegance” in biology, in which the methods we use to study or engineer life become simpler, more streamlined, and more capable over time. We continuously discover that “natural” creations, shaped by evolution over eons, can accomplish tasks in ways that human-made tools can still only dream of matching.
Gene editing is one example. Scientists used to employ laborious, brute-force techniques to alter DNA: random mutagenesis with chemical agents, perhaps, or splicing DNA with restriction enzymes. Then, we devised more targeted methods like zinc-finger nucleases and TALENs, which demanded skillful molecular tinkering for each gene target. And today, we have CRISPR, a system borrowed directly from bacterial immune defenses. All one needs to edit genes today is to modify a short RNA guide to direct a Cas enzyme toward a new target.
Or look at vaccines and the arc of immunization. As early as the 10th century, physicians in China relied on crude measures to protect people against disease. Variolation, for example, involved grinding up dried scabs from smallpox patients and then blowing them into a patient’s nostrils to stimulate immunity against the virus.
Today, researchers can instead encode an antigen’s blueprint in messenger RNA, deliver that message to human cells, and prompt those cells to “read” the encoded message and make the antigens. The immune system then recognizes the antigens as foreign, building memory cells that defend against future infections. Instead of crude methods (like snorting smallpox scabs), we can thus program the immune system directly. This is what I mean by the “slope toward elegance.”
In each of these examples, scientists happened upon an evolutionary ingenuity hidden in nature and converted it into a useful tool. And for me, that is the greatest wonder of all: There seems to be no end to biology’s marvels. The closer we look, the more wonders we find.
Niko McCarty is a founding editor of Asimov Press.
Cite: McCarty, N. “Seven Wonders of Biology.” Asimov Press (2025). https://doi.org/10.62211/21ut-33ds
Lead image by Ella Watkins-Dulaney.
Was Thomas unfamiliar with earlier studies of thermophilic microbes? After all, Alice Chien and colleagues had discovered Taq polymerase — an enzyme able to replicate DNA at high temperatures, which made modern polymerase chain reaction possible — in 1976, after isolating a microbe called Thermus aquaticus from a boiling Yellowstone geyser.
Written another way, for every nitrogen in a cell there are about four carbons, seven hydrogens, and two oxygens. A typical E. coli cell contains on the order of 1010 carbon atoms.