Why Nothing Can Grow on Mars*
Water is the most significant bottleneck in terraforming the Red Planet
(* = probably.)
Two years ago, a friend took me out to coffee in Boston and said, “I think we can engineer an organism to terraform Mars.”
Terraforming—transforming a planet so it can support life—has been the perennial dream of science-fiction authors and futurists, who, from Isaac Asimov to Kim Stanley Robinson, envisioned humanity inhabiting a multi-planetary civilization. Mars is the obvious candidate for this ambition because the other moons and planets in our solar system are even less hospitable.
The Moon has no atmosphere and minuscule supplies of water, carbon, and nitrogen, thus making it an unattractive target as anything more than a pit stop toward other destinations. Carbon on Venus (even diamond) is quickly oxidized into carbon dioxide by sulfuric acid and broiling temperatures. Saturn’s moons are somewhat more salubrious—Enceladus has liquid water, but no atmosphere and limited supplies of nitrogen—but are also far away. It would take a spacecraft seven months to reach Mars, but several years to reach Saturn.
There are many approaches to terraforming Mars, but first, one would have to overcome the inhospitable temperature. In 1923, a German physicist, Hermann Oberth, described giant space mirrors spanning hundreds of kilometers in diameter that could redirect solar radiation to warm a planet’s surface and make it habitable to astronauts. Another option might be to import greenhouse gases or aerosols to trap heat. But it would need to be a continual effort—greenhouse gases break down, and aerosol particles settle.
Modern synthetic biology offers plausible techniques by which we could terraform Mars. The feat could be achieved with something as direct as engineering a microbe that can survive on the Martian surface, and then letting it grow and spread over the planet. This is easier said than done, however. On Earth, life already thrives amongst radiation, toxins, and cold. But these “extreme” conditions tend to exist in isolated pockets and generally don’t converge. There are no known microbes that can survive all of these environmental assaults at once, which they would have to do to stay alive on Mars.
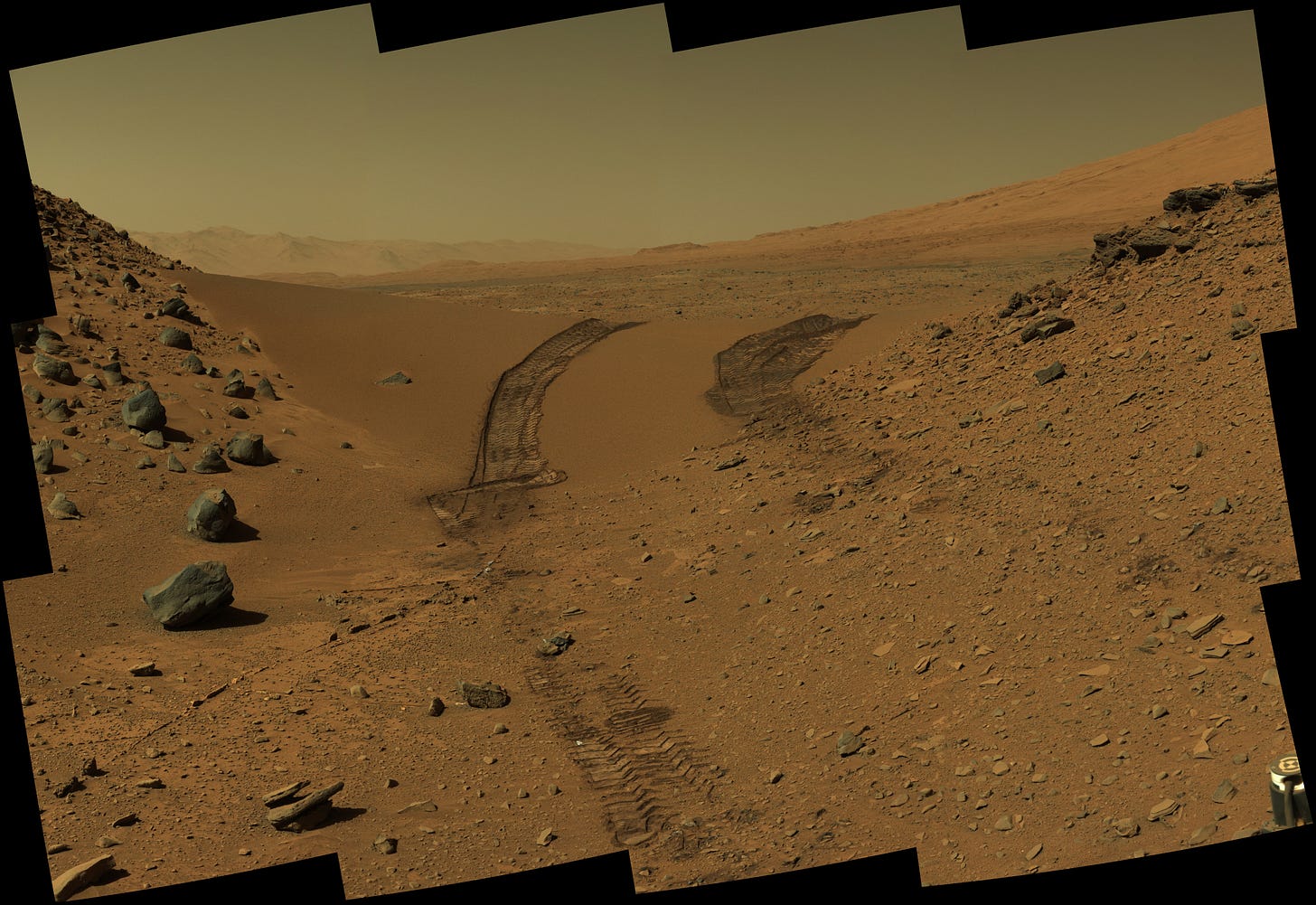
After that coffee meeting in Boston, my friend and I continued to imagine terraforming Mars. We researched the many “extreme” adaptations that lifeforms on Earth already possess and theorized how biotechnology could bring them together to make a microbe that thrived on barren, dry, toxic, and irradiated surfaces. Recently, we launched Pioneer Labs, a research nonprofit aiming to understand the true limits of life. Our goal is to make an organism that could grow outside on Mars. And our attempt—whether a success or failure—will improve the economics and capabilities of green biotechnology.
But first, some caveats. Even if we succeed in making a microbe capable of terraforming Mars, that doesn’t mean we’re necessarily going to send it to space. Our priority is to expand knowledge of the extreme limits of living organisms, not to unilaterally transform a planet or to sell terraforming microbes to the highest bidder. And, for what it’s worth, we don’t expect to succeed.
The surface of Mars is drier than almost any environment on Earth because of the low pressure, freezing temperatures, and high salt concentrations. Life on Mars would require adaptations that have never evolved on Earth. But if we did succeed, then our microbes could start changing Mars just as terrestrial microbes changed ancient Earth. They could create greenhouse gasses to warm the planet, crack open nitrates in the soil to thicken the atmosphere, and release oxygen for living things to breathe.
Progress would be slow, but turning the Red Planet green would be the only way for humans to walk freely on the Martian surface.
Martian Challenges
There are five primary obstacles that life must overcome to survive on Mars: radiation, toxins, temperatures, atmosphere, and water.
Radiation is often listed as the primary space hazard by astrobiologists. A trip to Mars and back would deliver about one-half sievert of radiation in the form of large, fast-moving particles that can penetrate even thick shielding. Five sieverts of radiation will kill about half of people exposed to it. Microbes can survive about one-thousand times more ionizing radiation than humans, though, which suggests that the ionizing radiation that NASA agonizes over when it comes to space missions is negligible for microbes.
What’s not negligible, though, is ultraviolet light. Mars doesn’t have much of an ozone layer, so despite the greater distance from the sun it gets a thousand times more UV than Earth does. The high-frequency UVC wavelengths are especially dangerous, and Mars gets between 3.2 and 5.5 watts per square meter of UVC. While astronauts are easily protected from UV by glass or a thin sheet of metal, microbes placed outside on Mars would be killed in mere minutes as the ultraviolet radiation shreds through DNA and destabilizes proteins that carry out important cellular processes.
Bacillus spores are considered by many biologists to be amongst the most durable lifeforms ever found. These spores can survive harsh conditions for years, with zero nutrients, and then “return to life” within minutes after germination. But they, too, are killed in minutes by UVC light at Martian levels.
Although UV radiation is clearly a problem for any potential terraforming microbes, other lifeforms on Earth have evolved clever mechanisms to resist its damaging effects. Deinococcus radiodurans, for example, is a walnut-shaped, radiation-resistant microbe that was first isolated in the 1950s. Arthur Anderson, a scientist in Oregon, was trying to sterilize canned meat with gamma radiation, but found living D. radiodurans cells in the meat even after subjecting it to incredibly high doses.
Later studies found that a lethal UV radiation dose for D. radiodurans is as high as 627 joules per meter squared. A metal-loving microbe from the Chilean desert (Hymenobacter) and a microbe isolated from an acidic volcano in Spain (S. solfataricus) can survive UV radiation at even higher levels.
In a way, this is surprising. No environments on Earth approach the levels of UV found upon the surface of Mars, and so there seems to be little reason for such high levels of radiation resistance to have evolved here. Perhaps the same molecular adaptations that allow these organisms to resist oxidants also work against UV.
Radiation-resistant microbes have evolved dozens of different molecular strategies that, together, compound to provide high levels of resistance. Some microbes make pigments that absorb radiation and antioxidants that protect against genetic damage, whereas others make multiple copies of the genome such that damage to one copy can be easily repaired by another.1
Toxins pose another threat to life on Mars, whose soil consists of high levels of perchlorate, a chemical found in rocket fuel and explosives. On Earth, perchlorates are considered industrial waste and are toxic to humans above 2 parts per billion, according to the Massachusetts Department of Environmental Protection. The perchlorate concentrations in Martian soil are high enough to prevent plant growth. Any liquid water on Mars is also expected to be saturated salty brine, with perchlorate concentrations ranging from 15-50 percent.
Microbes on Earth have evolved several strategies to deal with toxins. Debaryomyces hansenii, an unassuming species of yeast commonly found in cheese, can grow in broth with 30 percent perchlorate. The yeast cells synthesize lots of sugars to regulate their internal osmotic pressure and also attach sugars to proteins to stabilize them against damage caused by the perchlorates. Other organisms use perchlorate as an energy source, breaking it down into water and chloride ions and collecting energy from the reaction. NASA is collaborating with researchers at the University of California, Berkeley to exploit these organisms with the goal of detoxifying the Martian regolith so that crops can grow within the soil.
And then there’s the temperature. Mars, located about 50 million miles further from the sun than Earth, is exceedingly cold. The average surface temperature is about -60°C. While land at the equator can reach temperatures of 20°C, temperatures at the poles can plunge down to -150°C, cold enough for carbon dioxide to condense into dry ice out of thin air. Thermometers at the Vostok Station in Antarctica recorded the lowest temperature on Earth in July 1983, with a reading of -89.2°C.
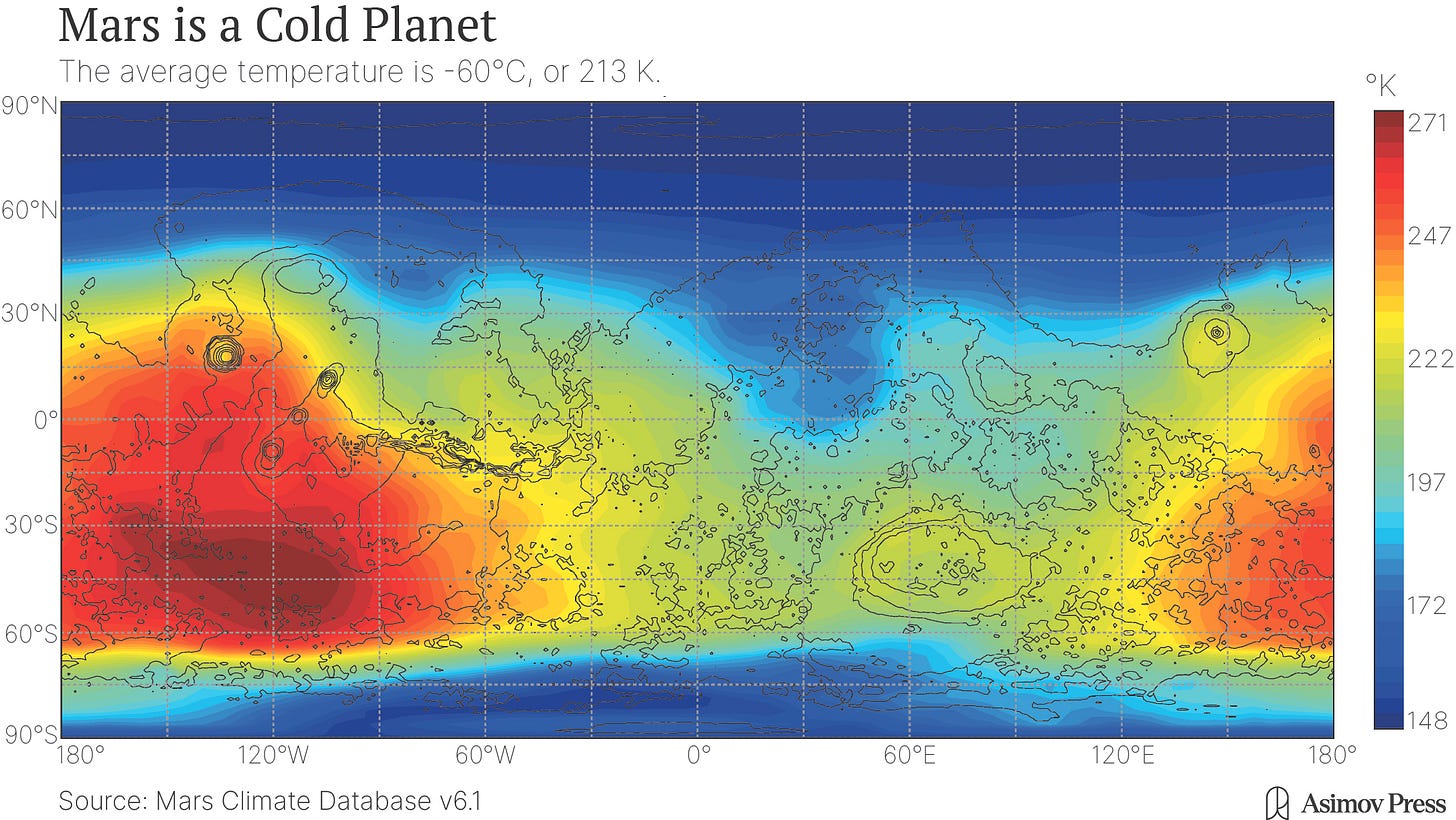
For a lifeform to thrive on Mars, it must first grow at temperatures below water’s freezing point. On Earth, a microbe found in arctic permafrost, called Planococcus halocryophilus, can grow in nutrient-dense media at temperatures as low as -15°C. A bacterium called Psychromonas ingrahamii, first isolated from glacial brine pockets, can grow at -12°C. At such low temperatures, these organisms only divide once every ten days, meaning it would take one gram of cells a full year to divide into 30 kilotons of biomass. Similar temperatures are found around the equatorial third of Mars, suggesting that there are already organisms on Earth that could withstand temperatures found upon a large portion of the Red Planet.
Withstanding the atmospheric pressure—or lack thereof—is another challenge. The Martian atmosphere is almost completely devoid of oxygen and about 1 percent as dense as Earth’s. It’s composed of 95 percent carbon dioxide, 3 percent nitrogen, and less than 2 percent argon, with small amounts of carbon monoxide and hydrogen. This mixture of gasses would kill most animals but is tolerable for microbes, many of which are anaerobic and thus either don’t require oxygen or are actively poisoned by it. The same perchlorate reducers that can withstand toxins are also mostly anaerobic, meaning they don’t live in places with oxygen. Cyanobacteria can grow well in a simulated Martian atmosphere, and high levels of carbon dioxide actually improve their photosynthesis rates.
At “sea” level, the atmospheric pressure on Mars is about 155 times lower than it is on Earth. But again, this is not a problem for microbial life. Bacteria can grow just fine at low pressures, so long as they can access the gasses required for metabolism, such as oxygen for aerobes, carbon dioxide for photosynthetic cells, and nitrogen for nitrogen-fixing microbes.
But even if a microbe could withstand all of these challenges—the low pressures, the biting temperatures, and the toxic soil—what of the water? There are some peculiar microbes on Earth that can survive on trace amounts of water that condense upon salt crystals, or that have evolved clever strategies to conserve water in times of scarcity. But all life on Earth requires liquid water for survival. Unfortunately, water is almost non-existent along the Martian equator, where temperatures are roughly similar to those found on Earth. Frozen water is available at the poles, where temperatures plunge to -100°C, or is found stuck to hydrated minerals. Scientists announced evidence of liquid water on Mars in 2015, but it's important to consider the state of that water; cold brines that are kept in a liquid state by extremely high concentrations of salt.
Water, and its lack of availability to lifeforms, would be the prevailing reason why nothing could grow on Mars.
Unavailable Water
If one dumped an entire lake onto Mars, some of the water would evaporate in the low-pressure atmosphere and condense at the frigid poles. Mars’ salty soil would absorb the rest, binding the water molecules tightly enough that they would be unable to evaporate.
It’s hard to overstate just how thirsty Martian soil is; salty and dry enough to suck water right out of any organism that has evolved on Earth. The entire planet is basically a desiccant, akin to those white packets found in packages of beef jerky that say “Do not eat.” Those little packets keep foods dry to prevent spoilage. Salting food, an ancient method of preservation that works by absorbing water so that it can’t be used by microbes, works in much the same way.
The habitability of Mars is restricted by the thermodynamic availability of its water, or its water activity. This scientific value is expressed as a number between zero and one, where one indicates pure water and zero means that there is no accessible water. Any water that is added to a material with a low water activity is quickly absorbed. Foods with a water activity of 0.6 or lower are basically immune to contamination because the lack of water prevents microbial growth. Dried fruits and honey have a water activity around 0.55, which means they will not spoil if kept properly dry. During the 1922 excavation of King Tutankhamun’s tomb, for instance, archaeologists discovered a jar of honey that was still edible.
The lower limit of water activity for existing lifeforms is 0.585. This record is held by Aspergillus penicillioides, a fungus that can live upon dust and dry paper. Only 12 known species of microbes can grow below a water activity of 0.7, and most of them are found in organic environments, such as honey.
All liquid water on Mars has a water activity below 0.5. There are no known lifeforms that could survive in this water, as the high salt concentration would quickly suck the liquid out of cells.
A 58-page NASA report from 2006 arrived at a similar conclusion. After “a deliberate and systematic search spanning several years,” the authors wrote, “Mars is either too cold or too dry to support the propagation of terrestrial life.” In reaching this conclusion, NASA assumed that the limits of life are temperatures above -20°C and a water activity of at least 0.5, both of which are permissive given the known biophysical limits of life on Earth.
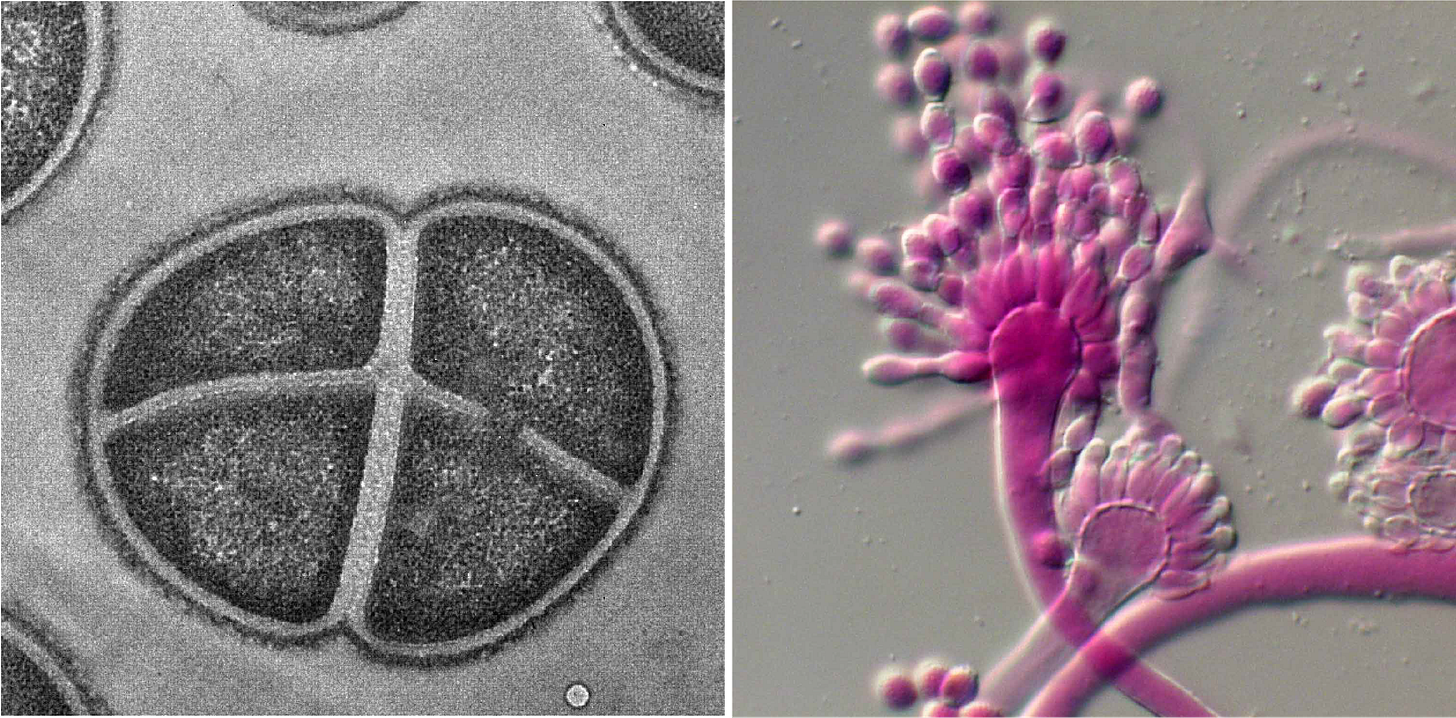
Unlike radiation and perchlorate, where there are no analogous conditions on Earth, there are environments on our planet with water activities below 0.5, and nothing grows there. Various dried foods are one example, but another is bittern salt ponds. If life has not figured out how to grow in such environments, even after billions of years of evolution, then water availability is likely a “hard limit” for Earth-based life.
However, not all hope is lost. An engineered organism, designed to terraform Mars, may not be able to grow in the perchlorate brines or low-water environments found on the planet, but perhaps it could make its own environmental niche that is more hospitable. One possibility is to engineer microbes that excrete insulating materials, trapping in heat and water and acting like a greenhouse to protect a small, microbial community from the raw Martian conditions. Biofilms already do this on Earth with sugar-based materials, but those substances would struggle to hold in enough heat or water. One would have to engineer microbes that produce insulation using novel nanotechnologies and materials designed to work at Martian pressures and temperatures.
Another organism from which we can draw inspiration is lichens, which are symbiotes of a fungus and an alga. Lichens can survive extreme conditions and can even absorb water from the air. A lichen found in Antarctica was once launched to the International Space Station. It spent 18 months on the outside of the space station, exposed to the harsh vacuum and unshielded solar UV, and mostly survived the ordeal.
Researchers later studied the same Antarctic lichen to learn whether it could live on Mars. Lichens are known to survive dry periods by drying out their cells and waiting to rehydrate once water returns. The symbiote continued to photosynthesize—using sunlight, water, and carbon dioxide to make sugars—under Martian pressures and temperatures. But the lichen didn’t grow.
Maybe there is a way to engineer lichens to extract water from the air. The Martian night is surprisingly humid, though the low total pressure means that a humidity of 100 percent on Mars is more similar to 10 percent humidity on Earth—about the same as Death Valley in the Mojave Desert on a summer day.
Ultimately, the lack of bioavailable water is the largest scientific barrier to Martian life, at least in the absence of human intervention. Unlike the organisms that can survive extreme radiation, toxins, temperature, and low pressure, there are no known adaptations that life on Earth has evolved to get around the water problem.
Near Futures
Life already survives many of the individual extreme conditions present on Mars. But no known organism can grow in the polyextreme environment that emerges when all of these challenges are rolled into one. By combining extremophilic traits found in organisms across Earth, we can learn more about how organisms might respond and adapt to such stressors, and what it might take to survive upon a modern Mars.2
Our goal at Pioneer Labs is to find the true limits of life, unshackled from the environments that exist on Earth. We plan to make a “polyextreme” microbe by endowing it with genes and pathways found in microbes that have evolved high resistance to UV radiation, temperatures, and so on. Then we’ll place the cells into simulated Martian environments and evolve them to turbocharge their adaptations to the harsh conditions. We’re not sure what we will find at the end of all this work. It could be that either Hymenobacter or D. radioduans radiation resistance is the best for Mars, for example, but most likely it will be a combination of the two.
This approach strives to create the most Mars-like microbe possible from existing Earth adaptations. But the final microbe, once engineered, is still unlikely to be able to grow on today’s Mars. Even so, we will probe the limits of the engineered cells to predict how much abiotic terraforming would be needed before the organism could be left to finish the job. And once we know how many space mirrors or tons of greenhouse gasses are necessary, we can start making a detailed plan to actually terraform Mars.
Even if we are successful in our scientific aims, there may be serious legal challenges to terraforming Mars with biology. NASA policy, seeking to prevent Earth microbes from destroying any possible Martian life forms before we have a chance to study them, prohibits releasing microbes in outer space. The Outer Space Treaty, to which all space-faring nations are signatories, contains a harmful contamination clause. This clause is primarily designed to prevent the spread of radioactive waste in space, but one could argue that releasing genetically engineered organisms to spread across a planet would be harmful.
Terraforming Mars to an Earth-like state might drive native Martian life (if it exists) extinct since our engineered organisms would invade and transform its habitats. We’ve spent several billion dollars searching unsuccessfully for life on the Red Planet, including $17 billion on landers and rovers, but it’s exceedingly difficult to prove the non-existence of a thing; there could, after all, be life on Mars. Terraforming the Red Planet is an irreversible decision that needs to be made by nations, not individuals.
Even if it proves impossible for anything to grow on Mars, engineered biology will be vital for supporting civilization in the stars. The development of polyextreme life at Pioneer Labs will reduce the investment required to manufacture therapeutics, food, structural materials, and chemical feedstocks vital to sustaining humans.3 This benefit will also extend to Earth, where much of the cost of biomanufacturing is wrapped up in catering to fragile organisms that require stable pH, temperature, and aeration conditions and can only grow on sterile and pure feedstocks.
In other words, Pioneer Labs is making a moonshot in humanity’s long quest to terraform Mars. Even if we fail, our home will be better because of it.
Devon Stork is a co-founder at Pioneer Labs and believes that biology is programmable nanotechnology. He received his Ph.D. at Harvard University in 2020. Reach him on Twitter @StorkDevon or by email at devon [at] pioneer [dash] labs [dot] org.
Cite: Devon Stork. “Why Nothing Can Grow on Mars.” Asimov Press (2024). DOI: https://doi.org/10.62211/43pf-11td
Microbes have evolved additional ways to protect against DNA damage, too. Some organisms accelerate the speed at which their proteins “turnover” to minimize damage or absorb metal into the cell to stabilize proteins. Others have evolved intricate strategies to repair DNA sequences shortly after they are damaged.
Our microbial engineering efforts include plans for biocontainment. We have outlined plans for a tiered sterilization strategy and will avoid using anything that is pathogenic. We also don’t expect engineered extremophiles to have a competitive advantage outside their niche in the wild any more than natural extremophiles do.
This field is known as in situ resource utilization. NASA has outlined plans to use local resources on Mars to convert atmospheric carbon dioxide to plastics and fuel, possibly by using minimally-shielded and easily constructed ‘greenhouses.’
I don't see you mentioning gravity. Early human development, even in the womb, relies quite a bit on gravity. I'm not sure that human children would develop normally in the 0.4g gravity of Mars. Healthy adult astronauts have significant health issues after a long stay in the ISS, even with all the exercise they do to retain muscle mass. So I guess even if we could terraform Mars by overcoming the hurdles mentioned in this article, I'm not sure a permanent human civilization could survive without some sort of artificial gravity.