Making Eggs Without Ovaries
It may soon be possible to make human eggs from stem cells, thanks to advances in a technology called in vitro oogenesis.
Today, we’re launching Issue 04 with this essay about the future of fertility and in vitro oogenesis — a technology that could soon be used to convert human skin cells into eggs or sperm — by one of its foremost developers. Thanks for reading.
In March 2023, a research team at Osaka University announced an extraordinary achievement: the production of viable eggs using cells taken from male mice.
Building on years of research into stem cells and reproductive development,1 the researchers, led by Professor Katsuhiko Hayashi, developed a method to induce male stem cells to lose their Y chromosome and duplicate the X chromosome, thus becoming female. From there, researchers took the female cells and grew eggs from them using an elaborate protocol they had initially published in 2016.
From the paper and ensuing media fanfare, a question emerged: When will this technology become available for people?
For nearly all of human history, there was only one way to make a baby. When a man and a woman love each other very much . . . well, you know the rest. But for many people, the usual method of baby-making doesn’t work. This is especially problematic for biological women, whose ovaries lose the ability over time to release healthy eggs. By age 40, most biological women are no longer able to reproduce.2 And in recent years, rates of infertility have increased along with the average age of childbearing.
The first method to circumvent some of the difficulties of conceiving a child, in vitro fertilization (IVF), came in 1978. During IVF, eggs are collected from the ovaries and mixed with sperm. As the fertilized eggs become embryos, they are transferred into the uterus. These basic methods have greatly improved in the last four decades, and more than 10 million people have been conceived using IVF. About 800,000 children are born from IVF every year. However, this technology still relies upon eggs that are formed naturally, which for many couples is simply not an option.3
But what if, as the Osaka team’s breakthrough in mice suggests, there was another way to get eggs? In the last decade, several researchers — myself included — have been exploring the tantalizing prospect of growing eggs in cell culture.
Such an approach would take cells from an adult4 — such as skin or blood — and reprogram them into induced pluripotent stem cells, or iPSCs. Much like embryonic stem cells, iPSCs have the ability to form any cell in the adult body; eggs included. Although generating human iPSCs is now routine, coaxing iPSCs to form eggs in a process known as in vitro oogenesis has only been successful on cells taken from mice.
Making eggs requires a precise series of developmental steps that are difficult to perform correctly in vitro, or in test tubes and cell culture dishes. It is for this reason that, despite a proof of concept in mice, human in vitro oogenesis has remained out of reach. Even with mouse cells, only about 1–3 percent of the eggs produced in vitro can form viable pups.
Still, researchers are working hard to better understand the steps required to form a healthy egg starting from a stem cell. If this technology could be replicated for humans, in vitro oogenesis would allow all kinds of people to reproduce who previously could not. But even without a complete method for in vitro oogenesis, solving some of these steps will open up intermediate technologies that could help treat infertility. In the coming years, people could have children from IVF at much later ages, for example, or cancer patients could restore their fertility after chemotherapy.
How Eggs and Sperm Get Made
In the laboratory where I work, just down the street from the bustling Fenway Park in Boston, there is an incubator with a 96-well plate inside. Each well in the plate contains an ovarian organoid, a clump of cells similar to a miniature ovary. To make the organoids, I differentiate human stem cells into germ cells, or egg precursors, and aggregate them together with ovarian supporting cells. These organoids are my little treasures. Every other day, I carefully feed them with growth medium over the several months it takes them to develop and form ovarian follicles. Right now, I’m testing different organoid culture conditions in an effort to re-create the developmental steps required for egg formation.5
From conception to puberty, several developmental steps happen inside the human body to create healthy eggs. The first step is germ cell specification, in which cells that go on to form eggs or sperm (the germ line) distinguish themselves from cells that form the rest of the body. About two weeks after conception, a small number of embryonic cells receive signals that coax them into primordial germ cells (PGCs), which are the progenitors for eggs and sperm. Male and female PGCs express similar genes and differ only in their sex chromosomes.
The first reliable protocols to differentiate human iPSCs into PGC-like cells in vitro were published in 2015. Although this process has become more efficient over the years, the fraction of cells that successfully become PGC-like varies from about 1 percent to 40 percent, depending on which batch of stem cells is used.
The second step is germline commitment, wherein, as you might expect from the name, the PGCs fully commit to forming eggs or sperm. After specification, PGCs migrate through the developing embryo to the gonads; ovaries or testes. There, they link up with gonadal supporting cells, called granulosa cells in the ovary or Sertoli cells in the testis. The male and female cells then begin to diverge into prospermatogonia or oogonia, respectively, dividing from a few dozen cells to a population of millions.
During this process, the cells’ genomes ditch their methyl groups, an important epigenetic mark. Epigenetic marks can be thought of as chemical “Post-it notes” on the chromosomes that control gene silencing and activation. Erasing methyl groups on DNA is necessary for normal egg and sperm development.6 The “wiped” cells then begin to express genes related to reproductive development and become receptive to signals for initiating meiosis, the process by which cells divide and split their chromosome numbers in half.
This is where things begin to get challenging, as it is immensely difficult to re-create the delicate interactions between germ cells and gonadal supporting cells in vitro. In recent years, however, researchers led by Mitinori Saitou at Kyoto University have made several important advances.
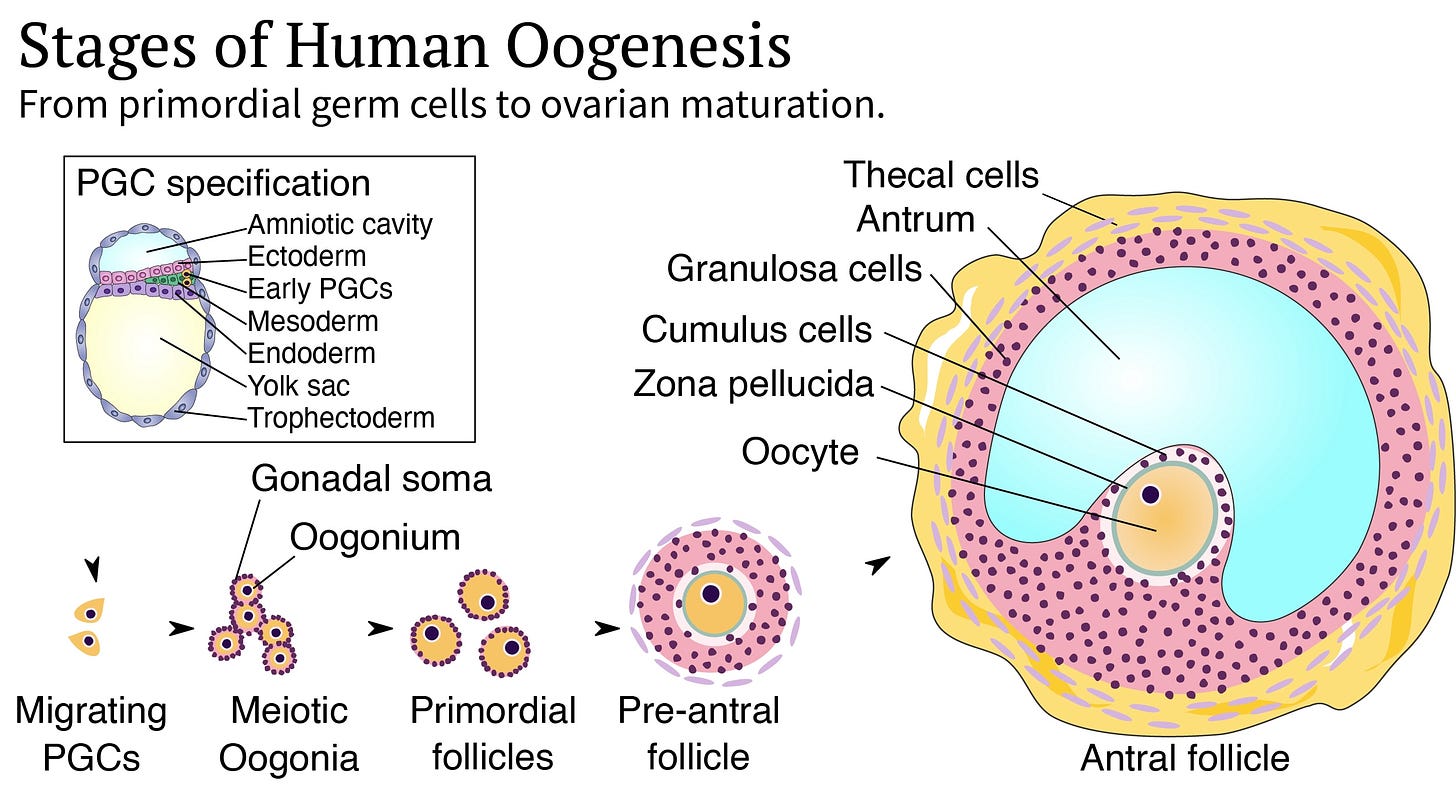
In 2018, Saitou’s team reported the first method to coax human PGC-like cells into the oogonia stage. They achieved this by treating mouse fetal ovaries with an enzyme mixture to break them apart into single cells, isolating the ovarian supporting cells, and mixing them with human PGC-like cells to form aggregates, which were then cultured in liquid media for up to 120 days. This multi-month method matches the duration of germline commitment in vivo.
Reaching the oogonia stage marked an impressive achievement in germ cell biology, because previous methods only went up to the PGC stage. More recently, the same lab published a related method that uses a specialized mouse cell line, instead of fetal ovaries, to support the development of human PGC-like cells to oogonia-like cells over several months.
However, it is possible to make oogonia-like cells from human stem cells much more rapidly. The most important step seems to be erasing DNA methylation. I have been working on a method to “fast-forward” through germ cell specification and commitment over a period of just five days. This method uses a chemical to inhibit DNA methylation, along with a genetic modification to activate expression of key transcription factors which regulate the expression of many other genes, inducing the cells to differentiate to an oogonia-like state.
After germline commitment, the third step to make eggs is meiosis, during which the chromosomes recombine with each other and then segregate to form haploid gametes (eggs or sperm) containing one copy of each chromosome. In males, meiosis doesn’t begin in the testis until puberty. Female meiosis begins during fetal development, but the immature eggs remain paused in meiosis for decades. So although the ovaries possess all the eggs they will ever have at birth, meiosis is not actually finished until after the egg is fertilized by sperm.
Up until now, the only way to achieve meiosis in the laboratory has been to culture germ cells, taken from a mouse, together with fetal ovarian supporting cells. As the cells grow, chemical signals activate meiosis in the germ cells. While promising in that it shows meiosis can happen outside of the body, to my knowledge, this technique has not been successful for human cells.
In our Fenway laboratory, I recently developed a method to directly initiate meiosis in human cells. Methyl groups attached to DNA are first artificially erased using a chemical inhibitor to mimic the erasure that happens during germline commitment. Then, I induce the signaling pathways involved in meiosis. Cells engineered in this way only progress part way through meiosis, but I’m steadily refining the method. If I successfully achieve complete meiosis, it would be a big step towards making eggs from adult human cells.
The fourth stage to make eggs is to grow the egg precursor cells to a large size. At roughly 0.1 mm in diameter, the egg is the largest human cell. It contains stockpiles of proteins and RNAs that are used by the developing embryo. Eggs grow inside ovarian follicles, where granulosa cells pump them full of nutrients. During this process, the follicle also grows, reaching up to a few centimeters in diameter.
This step in egg development has not yet been achieved using human stem cells. However, ovarian follicle biopsy samples can be transferred into cell culture. And even when they are removed from the body, the ovarian follicles continue to produce mature eggs. But this process is inefficient and, as of April 2024, no live births have yet resulted. Still, methods to culture follicles in the laboratory are a promising option for preserving fertility for cancer patients whose ovaries are removed. These methods could also be applied to ovarian tissue derived from human stem cells once scientists figure out how to reach the primary follicle stage, where the egg cells have completed the first stage of meiosis but have not yet grown large enough to form embryos.
Interestingly, it is possible to skip over meiosis and make large egg-like cells directly from mouse stem cells. But cells made in this way don’t typically carry the correct number of chromosomes, and therefore the eggs don’t develop normally after fertilization.
The final step for egg development is establishing proper epigenetics. As an embryo develops, these epigenetic marks could, for example, control the signaling genes that tell the placenta how much to grow. After being erased during germline commitment, epigenetic marks must be properly re-written during egg development. But even in mice, the only species where in vitro oogenesis has succeeded, 97–99 percent of the eggs produced are not able to give rise to living offspring. Poor epigenetics is a major reason for this low efficiency.
In theory, an in vitro oogenesis method that exactly mimics natural ovarian development would be able to achieve the proper epigenetics without additional intervention. But mimicking natural human ovarian development would take, at minimum, several years, and the natural process of human oogenesis isn’t even particularly efficient! Even in a woman’s years of peak fertility, the chance of one of her eggs developing properly after fertilization is only about 30 percent.
Some of this low success rate is due to errors during meiosis, although incorrect egg epigenetics also accounts for the failure of many embryos to develop properly. For example, a recent study on human embryos found differences in egg-derived DNA methylation between healthy embryos and embryos which failed to activate important developmental genes.
Thankfully, epigenetic engineering has the potential to make in vitro oogenesis faster and more efficient. Scientists have been developing methods for precisely erasing and writing epigenetic marks, including DNA methylation and histone modifications, by using CRISPR technology to guide epigenetic modifying enzymes to particular sites in the genome.
Epigenetic CRISPR tools are targeted to a particular DNA sequence using a guide RNA molecule. But instead of cutting the DNA, as CRISPR-Cas9 normally would, epigenetic editors add or remove chemical tags. By adding and erasing methylation at important regulatory sites in the genome, scientists were able to create mice with both sets of chromosomes originating from a naturally-formed egg, which is usually impossible due to epigenetic imprinting. Although large-scale engineering of the epigenome remains difficult, recent advances in multiplex CRISPR editing, which could be adapted for epigenetic editing, make me optimistic that this final step in egg development could be recreated in the laboratory.
Similar technologies might also be used to make sperm from stem cells. Infertility affects roughly 7 percent of men, and about 1 percent of men lack sperm entirely. But compared to oogenesis, in vitro spermatogenesis has received much less attention for two reasons.
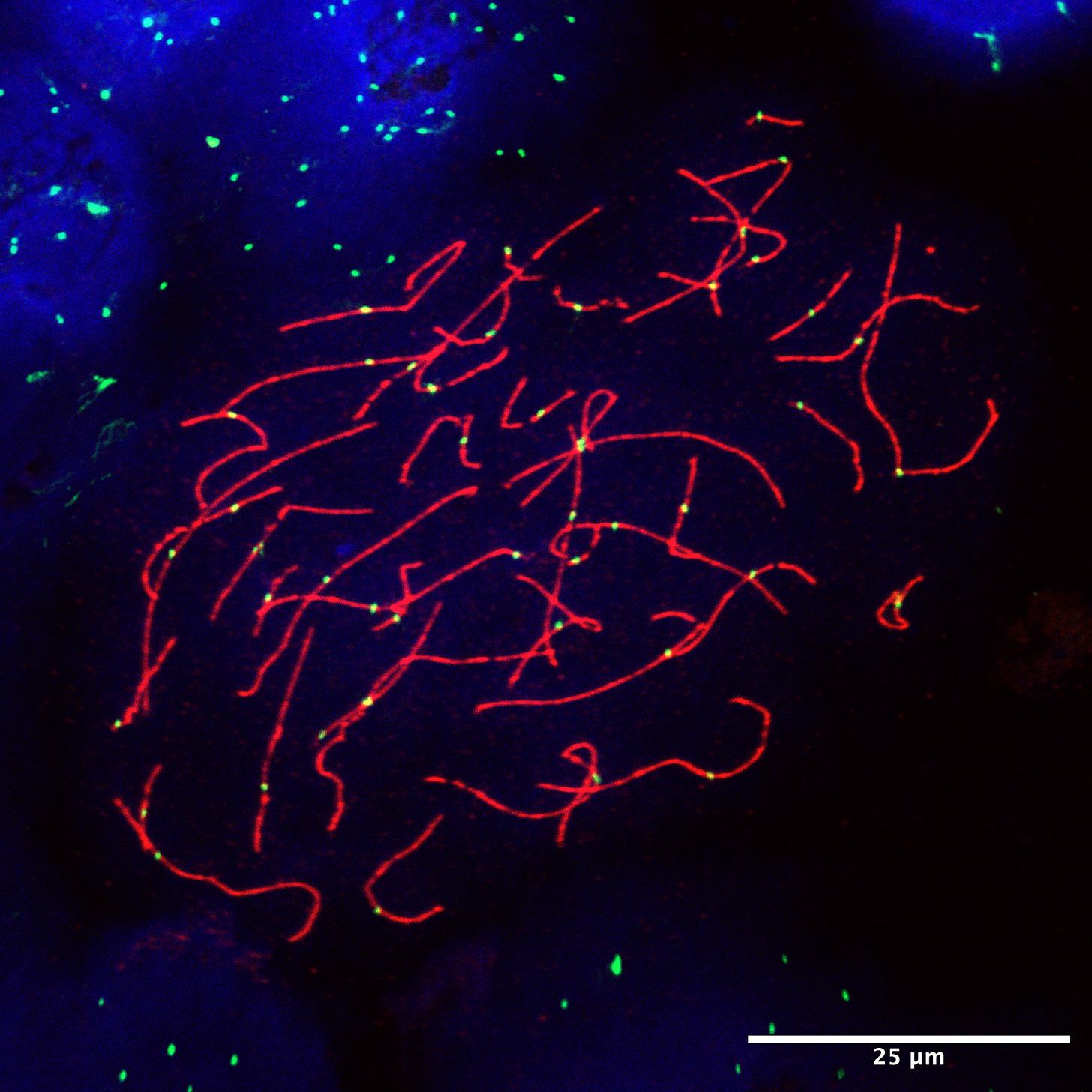
First, sperm are much more readily available than eggs. Although sperm production declines with age, older men can still reproduce naturally with younger partners or egg donors.7 Second, spermatogenesis is more complicated than oogenesis. Whereas ovarian follicles are small, self-contained structures, sperm are produced inside seminiferous tubules. There are roughly 600 meters of these thin tubes, each one-fourth the diameter of angel-hair pasta, coiled inside each testis of every man.
In the laboratory, ovarian follicles can be made simply by mixing together ovarian supporting cells and germ cells. But this doesn’t work for testicular organoids because a random aggregate of supporting cells and germ cells lacks the long-range organization required to form seminiferous tubules. It would be like boiling a hunk of bread dough in tomato sauce, and expecting it to make spaghetti. Mimicking natural sperm development outside of the body will therefore require significant advances in organoid technology.
Artificial spermatogenesis may be possible inside the human body, however. Unlike the ovary, the adult testis contains a self-renewing population of stem cells, called spermatogonial stem cells (SSCs), which are the precursors to sperm. Because chemotherapy often kills SSCs, cancer researchers have been exploring the possibility of preserving SSCs and transplanting them back into a patient after treatment. A team of researchers primarily based at the MD Anderson Cancer Center in Texas recently demonstrated this procedure in rhesus monkeys, successfully restoring their fertility after irradiation.
If human SSCs can be derived from iPSCs, then those cells could be transplanted into a patient’s testis.8 Although this method would require a pre-existing testis, it would still be useful to treat many cases of male infertility. For example, non-obstructive azoospermia, a condition affecting one percent of men, makes it so the testes are not able to produce sperm, often due to mutations that cause SSCs to be absent or defective. Treatment for azoospermia might involve editing patient-derived iPSCs to correct mutations, then differentiating the iPSCs to SSCs and transplanting them back into the patient.
Reaching the Clinic
The first announcement of a human egg grown from a stem cell may be only a few years away. However, initial methods to do so are unlikely to result in high-quality eggs suitable for making healthy babies. Although quantity may have a quality of its own when it comes to growing eggs in a research context, this is definitively not true for human reproduction.
Recall that the first demonstrations of in vitro oogenesis from mouse cells resulted in just 1–3 percent of eggs capable of forming viable pups. Lab-grown eggs often have epigenetic issues and, because nondestructive epigenetic testing of embryos is difficult, using in vitro grown eggs for reproduction currently poses an unacceptable risk of making a baby that suffers from an epigenetic disorder, such as Angelman syndrome.9
Before full in vitro oogenesis becomes feasible, we are likely to first see assisted reproductive technology helping to get the final stages of egg development “over the finish line.”
A common problem during IVF is that many collected eggs are not fully mature and are therefore unsuitable for fertilization. Gameto, a New York City-based startup company, has recently begun clinical trials in which immature eggs collected during IVF are cultured with ovarian-supporting cells derived from human stem cells. This process is intended to help the immature eggs reach a more mature stage. Although this technology may only increase the number of embryos that can be produced per IVF cycle by about 25%,10 this is still an important benefit for couples trying to conceive.
The next technology to see clinical use will likely be growing mature eggs from earlier precursors found in ovarian biopsy samples, a process known as in vitro follicle growth and maturation. Taken to an extreme, this would look similar to Aldous Huxley’s vision in Brave New World, in which ovarian tissue samples are used to grow batches of eggs by the thousands.11 Less speculatively, this technology could be used to restore fertility in biological females who have lost most, but not all, of their healthy ovarian follicles.
Several cancer patients have already been able to restore their ovarian function after chemotherapy, and even have healthy babies, by re-transplanting a frozen ovarian tissue sample taken before chemotherapy back into their ovaries. This shows that the ovarian follicles can be preserved outside of the body, and currently, in vitro culture of ovarian tissue is almost ready for clinical use. The main bottleneck just seems to be recruiting enough patients for clinical trials. So far, this technology has been developed focusing on cancer patients, not healthy women, which makes trials much more difficult to run.
But for many people, starting with a piece of ovarian tissue is not an option. And this brings me back to in vitro oogenesis, and existing barriers to its development.
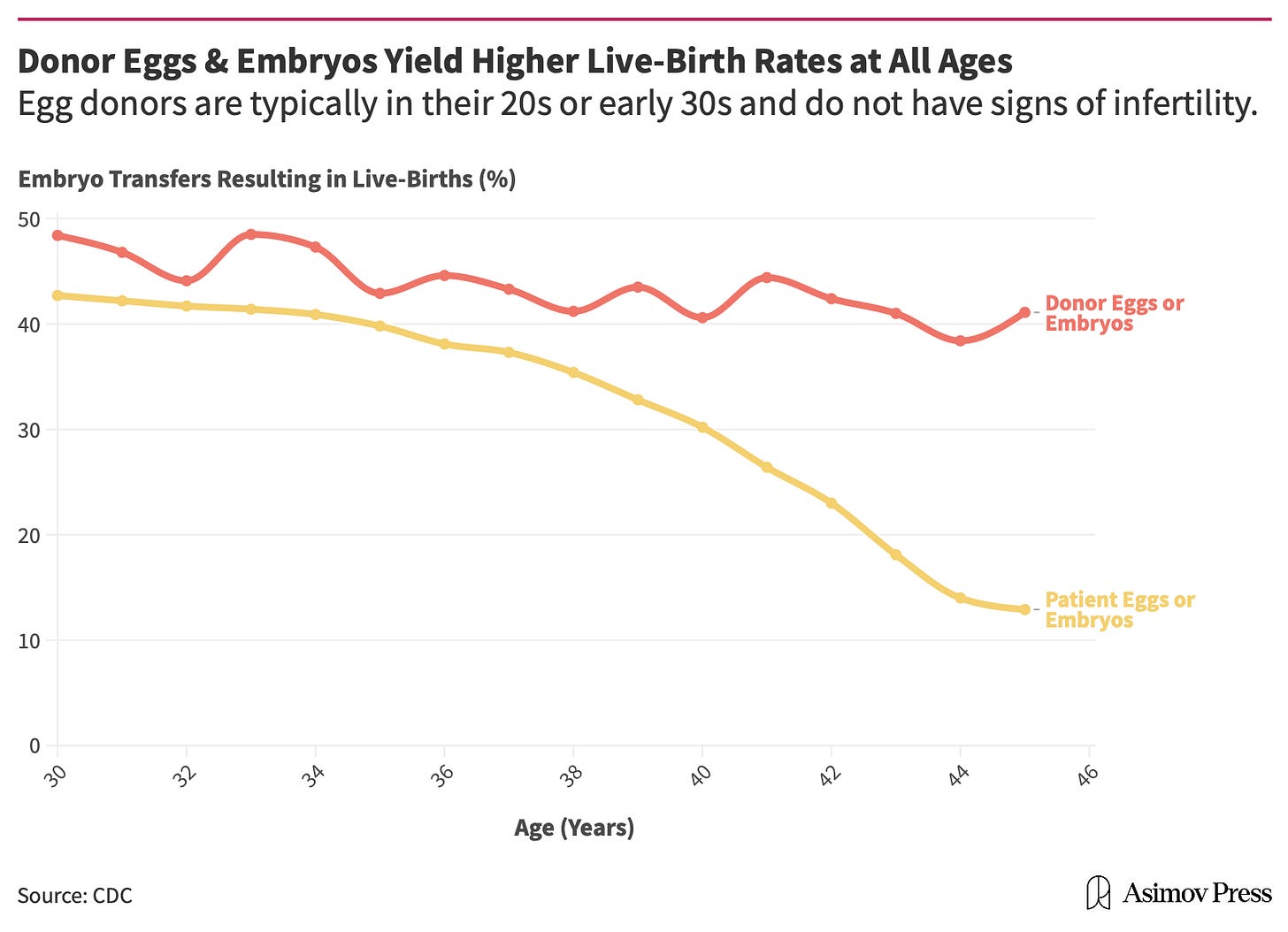
When the first birth of mice from lab-grown eggs was announced in 2016, researchers around the world earnestly began working to translate the method to humans. But this has proven challenging for multiple reasons. The original mouse protocol used fetal ovarian cells to support the stem cell-derived germ cells, but human fetal ovarian tissue is both ethically and technically impractical to obtain. Mouse fetal tissue can support the development of human oogonia to a limited extent, but it’s not suitable for full oogenesis.
Human and mouse cells also have several differences in gene regulation. Culture conditions optimized for mouse cells do not work well for human cells.
And finally, human development is intrinsically much slower than that of mice. Starting from the embryo, it takes approximately 15 years for the human ovary to fully develop, compared to 9 weeks for the mouse ovary. Human development can be sped up considerably in cell culture, but even so, experiments regularly take several months, making it hard to iterate quickly.
Nonetheless, the pace of progress in the field, especially by the researchers at Kyoto University, has made me hopeful that the first in vitro-grown human eggs are only a year or two away. Several startups, most notably Conception, Ivy Natal, Dioseve, and Vitra Labs, have also been toiling away at this problem and may be close to solving it. But this will merely mark the end of the beginning.
Judging from the results with mice, the egg quality from in vitro oogenesis in human cells will initially be very low, likely because of errant epigenetic marks. It may be possible to transfer chromosomes from a low-quality egg grown in vitro into a higher-quality donor egg,12 but this would place further demands on the already limited supply of donated eggs and would not fully fix the epigenetic problems. Extensive safety trials, including the birth of healthy non-human primates, will be required before the first human clinical trials can begin.
In the more distant future, eggs grown in vitro will be of a higher quality than natural eggs because culture conditions in a laboratory can be controlled and optimized to a degree not possible in natural ovaries. In the near term, the opposite will hold true; babies born from eggs grown in vitro will likely face an increased risk of developmental disorders. This brings up a perennial and hotly debated ethical question: how much risk is acceptable?
It is clearly unethical to make a baby that will experience mostly suffering during its lifetime. If 25 percent of babies born from in vitro-grown eggs experience painful deaths, then surely most people would be appalled. Even so, it is not illegal for two carriers of Tay-Sachs disease to reproduce naturally, despite the fact that their babies would face similar odds.
After confronting the consequences of coercive eugenics in the last century, society has rightly decided that it is unacceptable to prohibit people from reproducing. So if otherwise infertile individuals want to have biological children using a risky technology, should they be denied?
This question will fall to the FDA and similar medical regulatory agencies in other countries.13 The FDA has adopted a relatively lenient regulatory approach toward reproductive technologies, but U.S. politicians have previously mandated that the FDA ban trials of promising reproductive technologies, including a technology called mitochondrial replacement therapy14 that is used to make embryos with chromosomes from a man’s sperm and a woman’s egg, together with mitochondria from the egg of a second woman.
Mitochondrial replacement therapy has been used successfully in other countries in cases where a woman has a mitochondrial disorder. As new technologies become more widely appreciable, their regulation may become a hot-button political issue. After all, even IVF, well-established as it is, is under attack these days in the United States.
Costly Prospects
Should oogenesis technology emerge even against the backdrop of contentious politics and stringent regulation, there remains the question of who will be able to access it and what exactly it will be good for. Although demographers have long worried about declining fertility rates, in vitro oogenesis is unlikely to reverse this trend.15 Reproductive decisions are mainly based on social and economic factors, such as the cost of housing and childcare. Although I aspire to make in vitro oogenesis accessible to everyone regardless of wealth, it is likely to be quite expensive in the near term.
IVF costs $10-20,000 per cycle in the United States and is considerably less technically challenging to perform than in vitro oogenesis. A company called Viagen Pets charges $50,000 to clone a cat, in a process that is not only much easier than growing an egg but also in lower demand. CAR-T cancer therapy, a personalized therapy that involves culturing and engineering a patient’s cells, costs $370,000–$530,000 in the USA, although the price is only $30–$40,000 in India.
Based on the costs of these procedures, I expect the first commercially available in vitro oogenesis therapy to cost around $150,000–$250,000, not including additional costs to fertilize and implant eggs after they are grown in vitro.
Instead of starting a second baby boom, new reproductive technologies will mainly expand the kinds of people who are able to have biological children. First, growing eggs from ovarian biopsy samples will allow women to obtain eggs even when their ovarian reserve is diminished. This could extend the age of fertility into the mid-40s.
Furthermore, this technology would allow younger women to grow large numbers of eggs from tissue samples. By enabling women to freeze more eggs, they would have a better chance of having babies later.
With in vitro oogenesis, the possibilities of reproduction will expand even further. Older women, trans women, and men could all grow eggs using their genetic material, although this will be most difficult for biological males. And because parents who currently select embryos to implant based on positive polygenic traits (such as a low cancer risk) have a limited number of embryos to choose from, growing large numbers of eggs would also moderately increase the potential gains of embryo selection.16
Over time, new reproductive technology will slowly but steadily transform everything from demographics to the way we think about choice. Tomorrow’s adults are today’s babies, and twenty years from now, the question of “where do babies come from?” might have a wide variety of answers.
In my lifetime, I hope to see a world where anyone who wants to can have biological children.
***
Metacelsus is a PhD student who researches synthetic biology, stem cells, and reproductive development. He also writes the blog De Novo.
Header image by Ella Watkins-Dulaney, PhD.
Cite: Metacelsus. "Making Eggs Without Ovaries." Asimov Press (2024). DOI: https://doi.org/10.62211/41pr-87er
Disclosure: The author has previously received research funding from Gameto, and currently receives patent royalties from Gameto. The author is also the founder and CSO of Ovelle, a startup company developing in vitro oogenesis technologies.
As Reviewer 1 wrote, “Most of the approaches described are not so novel from a technological perspective . . . However, adding these approaches together to achieve egg production from male mice is clearly a major achievement.”
Even with IVF, the chance is only 44 percent after three cycles.
This includes couples of two biological males, or couples in which a woman is infertile due to a lack of healthy eggs.
Skin cells and blood cells are the easiest cell types to access, but for safety purposes, it would be best to choose a starting cell type with a low rate of mutations, such as a bone marrow stem cell.
Finding the right conditions is quite tricky. Once, I accidentally caused my cells to make cardiac tissue instead of ovarian tissue, and the organoid started beating!
Aside from epigenetic imprinting, several important genes for egg and sperm development will only be expressed if the DNA in their promoters is unmethylated.
Famously, U.S. President John Tyler (born in 1790) still has a living grandchild, due to men in that family having children late in life.
A few papers in the 2010s claimed to derive human SSCs from iPSCs, although not convincingly.
Epigenetics vary considerably between the trophectoderm, which forms the placenta, and the inner cell mass, which forms the actual baby. Trophectoderm biopsies, which are commonly used for genetic testing, are much less useful here, because they don’t sample the inner cell mass, and therefore may not detect epigenetic abnormalities in the inner cell mass. But sampling the inner cell mass would damage the embryo, so there’s not an easy way out of this problem.
Typically 15 - 25% of eggs retrieved for IVF are immature (GV stage), but in egg collection protocols that use lower hormone doses to minimize side effects, this percentage can be much higher.
Referred to as “Podsnap’s Technique”, pg. 6.
This is similar to what is currently done in mitochondrial replacement therapy, wherein chromosomes from one egg are transferred to another.
Under 21 CFR 884, the FDA regulates assisted reproductive technology under the regulations for medical devices, which are not quite as strict as the regulations for drugs.
The FDA website announcing the ban also says that mitochondrial replacement therapy “introduces a genetic modification,” but this is straight-up incorrect. Neither the mitochondria nor the chromosomes are edited or modified. Instead, chromosomes from an egg with unhealthy mitochondria are merely transferred into an egg containing healthy mitochondria.
Artificial wombs would, but these are orders of magnitude more difficult to achieve, for reasons that will be covered in a forthcoming article in Asimov Press.
The expected gain is proportional to the square root of the logarithm of the number of embryos, so you would need to select from 10,000 embryos to achieve twice the gain of selecting from 10 embryos. However, performing multiple rounds of in vitro gametogenesis would allow for multiple stages of selection, leading to larger gains.
This will change everything. Thank you for explaining where the technology is going. IVF is not the end game solution to infertility
Great article. Kudos for writing this!