Day Zero Antivirals for Future Pandemics
Medicines modeled off the innate immune system have the best chance of protecting us during the next viral outbreak.
Brian Wang (co-founder of the nonprofit Panoplia Laboratories) outlines his approach to making broad-spectrum antivirals. He also explains how they could be used to combat pandemics on “day zero,” well before vaccines are developed.
Ebola tore through Western Africa in 2014, killing an estimated 11,325 people in two years. After the outbreak ended, it took another three years for the first Ebola vaccine by Merck to be approved. When COVID-19 emerged in 2019, by contrast, mRNA vaccines developed by Pfizer and Moderna took just 326 days from the initial sequencing of the virus to gaining approval for emergency use.
Timelines for vaccine development are shrinking, but can it move even faster? The Coalition for Epidemic Preparedness Innovations (CEPI)—a nonprofit organization that funds vaccine research and development—thinks so. In 2022, they raised $2 billion from private investors, nonprofit organizations, and over a dozen national governments to advance their mission to deliver pandemic vaccines within 100 days.
Even this aggressive target of 100 days won’t always be fast enough to slow a pandemic, however. The Omicron variant of COVID-19 spread with alarming rapidity; after China ended its zero-COVID policy in 2022, estimates suggest Omicron infected upwards of 80 percent of its population—over a billion people—in about one month.
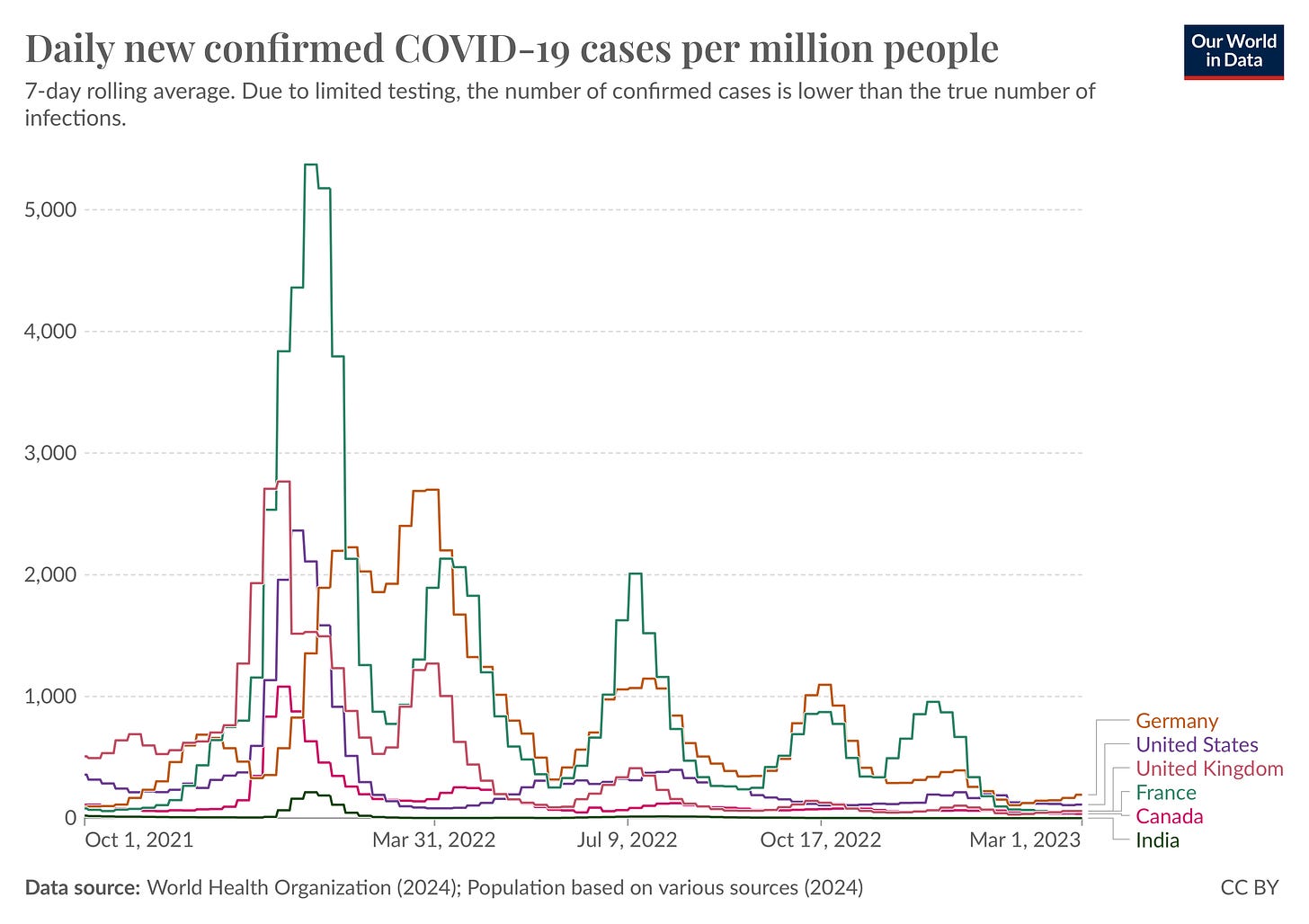
Fortunately, vaccines aren’t the only way to slow the spread of pathogens. We can also deploy broad-spectrum antiviral drugs, which treat infections caused by multiple viruses before vaccines are developed. These drugs would be ideal tools to bridge the wait for a vaccine against a quickly-spreading virus. And ideally, such broad-spectrum antivirals would be deployed at the earliest signs of an outbreak, thus enabling a pandemic response to begin on “day zero,” rather than day 100.
Broad-spectrum antivirals have been somewhat of a disappointment so far. Remdesivir, a decade-old broad-spectrum antiviral developed by Gilead and previously tested against Ebola, was quickly approved for emergency use against COVID-19 in May 2020, seven months earlier than the first mRNA vaccines. Lengthy manufacturing timelines slowed its deployment, though; it wasn’t until October—five months later—that drug manufacturers scaled up enough to meet national demand. Nor was remdesivir as effective as people hoped. One large-scale clinical trial found that it only reduces the relative risk of death by about ten percent in hospitalized patients.
We need to make more effective and rapidly deployable broad-spectrum antivirals. For guidance on how to do so, we can learn from the world’s most experienced broad-spectrum antiviral developer: the innate immune system.
As our first line of defense against infection, the innate immune system includes the skin that covers our body, the mucus that lines our organs, the immune cells that patrol our tissues to gobble up pathogens whole, and the antiviral molecules inside our cells that disrupt viral replication. Understanding the principles that make these tools so effective, and adapting them to make new types of broad-spectrum antivirals, may be the best path toward building better day zero medicines for the future.
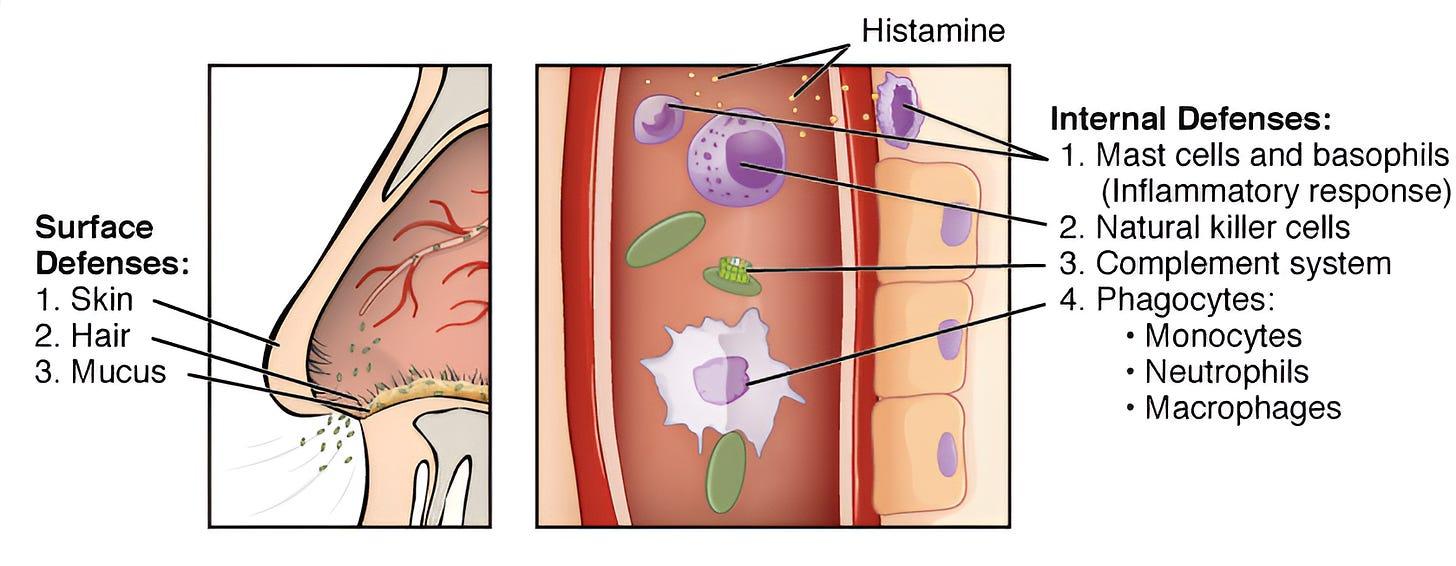
The innate immune system has been evolutionarily successful for three reasons: its tools target the most fundamental properties of viruses, act as prophylactics—meaning they prevent diseases, rather than treat them—and are widespread.
Consider mucus, which resembles a dense spider web lining the surfaces of our body’s internal organs, trapping viruses before they can infect the underlying cells. Because mucus targets a fundamental property of viruses—that they must physically reach host cells in order to infect them—almost all viruses are hindered by it. Mucus is also effective because it’s prophylactic; it prevents cells from becoming infected in the first place rather than attempting to curb infection after viral replication has spiraled out of control. And finally, it is widespread: cells all along the respiratory, digestive, and reproductive tracts constantly produce this viscous substance, such that viruses are likely to encounter mucus no matter their portal of entry.
Our next generation of broad-spectrum antivirals should aspire to these mucus-like qualities. They should target fundamental properties of viruses, they should be prophylactic such that they are effective against the next pandemic virus regardless of its identity, and they should be widespread so that we can immediately pull them off shelves at a pandemic’s onset.
This work is already underway. Many research groups are now making broad-spectrum antivirals inspired by the innate immune system, leveraging recent advances in chemistry and biology. Last year, several colleagues and I joined these efforts by co-founding Panoplia Laboratories, a nonprofit organization developing broad-spectrum antivirals using tools from synthetic biology. If any of us succeed, we’ll be better prepared to combat the next pandemic starting on day zero.
Antiviral Limits
Estimates suggest that more than 600,000 different viruses could infect humans, most of which are still unknown. For this reason, developing broad-spectrum antivirals presents an enormous scientific challenge.
Existing medicines only pose a partial solution. Remdesivir and other broad-spectrum antivirals work by jamming up RNA-dependent RNA polymerase (RdRp), an enzyme that nearly all RNA viruses use to replicate their genomes. However, the structure of RdRp differs between viruses, such that many are relatively unaffected by these molecules. Remdesivir substantially reduces the ability of Ebola virus, coronaviruses, and RSV to infect cells, but fails against Chikungunya virus, influenza, and some hepatitis viruses.
Rather than target viral proteins, then, some scientists are designing antivirals that target more fundamental properties of viruses. In 2012, a research group at MIT isolated the major components of mucus from pig stomachs (“porcine gastric mucins”) and tested its ability to inhibit infections caused by human papillomavirus, influenza virus, and Merkel cell polyomavirus.
Cells that were covered in a one percent mucus solution had ten-times fewer infections compared to control cells covered with a standard buffer. By following fluorescently labeled virus-like particles under a microscope, the scientists also observed how the mucus matrix immobilized virus particles. Adding these mucins to hygiene products, such as mouthwash and toothpaste, could provide regular boosts of antiviral protection.
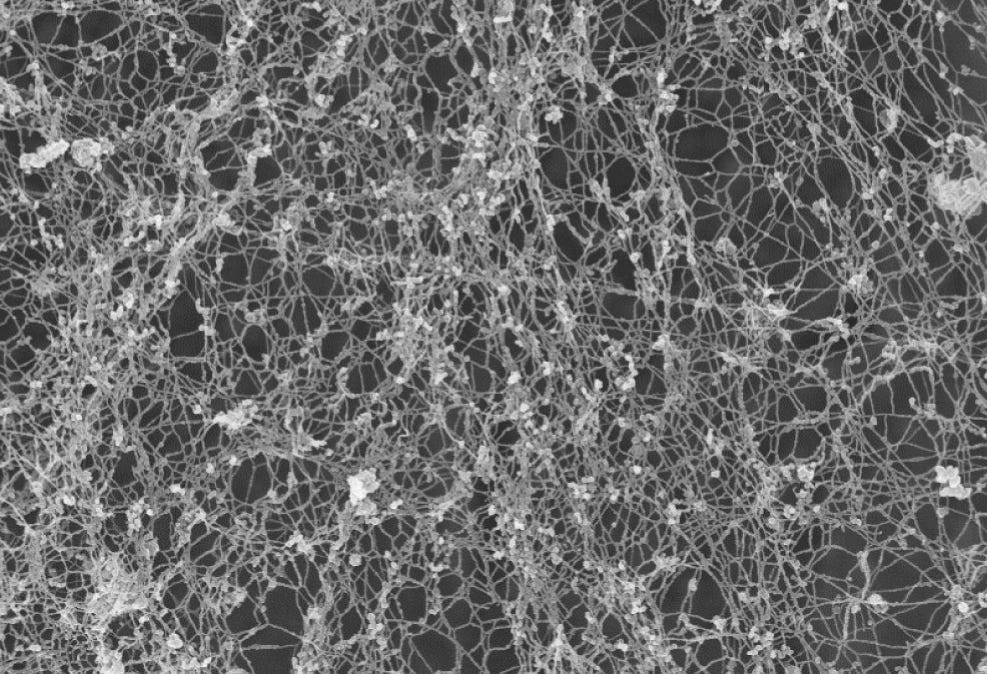
Other efforts to build broad-spectrum antivirals have focused on another conserved feature of viruses: double-stranded RNA. While human cells have low levels of double-stranded RNA, most viruses produce double-stranded RNA as part of their replication cycle, suggesting it may be a promising target for broad-spectrum antiviral development. In fact, the innate immune system uses double-stranded RNA as a key signal that an infection is underway.
In 2011, research groups at the MIT Lincoln Laboratory and Boston University fused together two different proteins to make broad antivirals: one protein binds to double-stranded RNA, and the other triggers apoptosis, or cell death. These engineered proteins enter cells and act like a quick-trigger suicide pill: if a cell were to be infected by a virus, the presence of double-stranded RNA triggers the cell to apoptose, killing the cell and cutting off the virus’s ability to use that cell’s resources to replicate. If this happens across enough cells, the viral infection burns out. Both groups showed that these proteins—dubbed DRACOs and dsCAREs, respectively—reduced the replication of viruses across ten separate viral families in cells and across three separate viral families in mice.1
Promising preclinical data alone isn’t enough to produce a day zero antiviral, however. We need to demonstrate real-world clinical efficacy. And regrettably, one does not guarantee the other. After all, remdesivir was effective against coronaviruses in lab studies but underperformed clinically. If broad antivirals are ever going to progress through testing and succeed in human patients, they must first overcome specific challenges related to timing.
Prophylactics
After a viral infection takes hold, the viruses infect cells, replicate, and grow exponentially in number. One infected cell typically makes 10 to 100 more virus particles that go on to infect other cells. Within days, the number of virus particles in our body balloons to billions and we begin to feel sick. For COVID-19 and influenza, the peak viral load often falls on or around the day of symptom onset. Then, the amount of virus declines as our immune system catches up. Symptoms caused by mild infections resolve shortly after the virus is cleared from our body. If we’re unlucky, however, our immune system goes into overdrive even as viral load subsides, causing damage to our lungs and sending us to the hospital.
This exponential infection dynamic poses a challenge for existing broad-spectrum antiviral treatments. Since these antivirals work by disrupting viral replication, it’s best to treat patients while the virus, and not the immune overreaction, is responsible for continuing symptoms. But by the time a patient receives treatment—perhaps not until a week after symptom onset, as was the case early in the COVID-19 pandemic when only hospitalized patients were eligible for remdesivir—viral load may already be on its way down. Starting antiviral treatments after the viral load “peaks” inherently limits their medical efficacy.
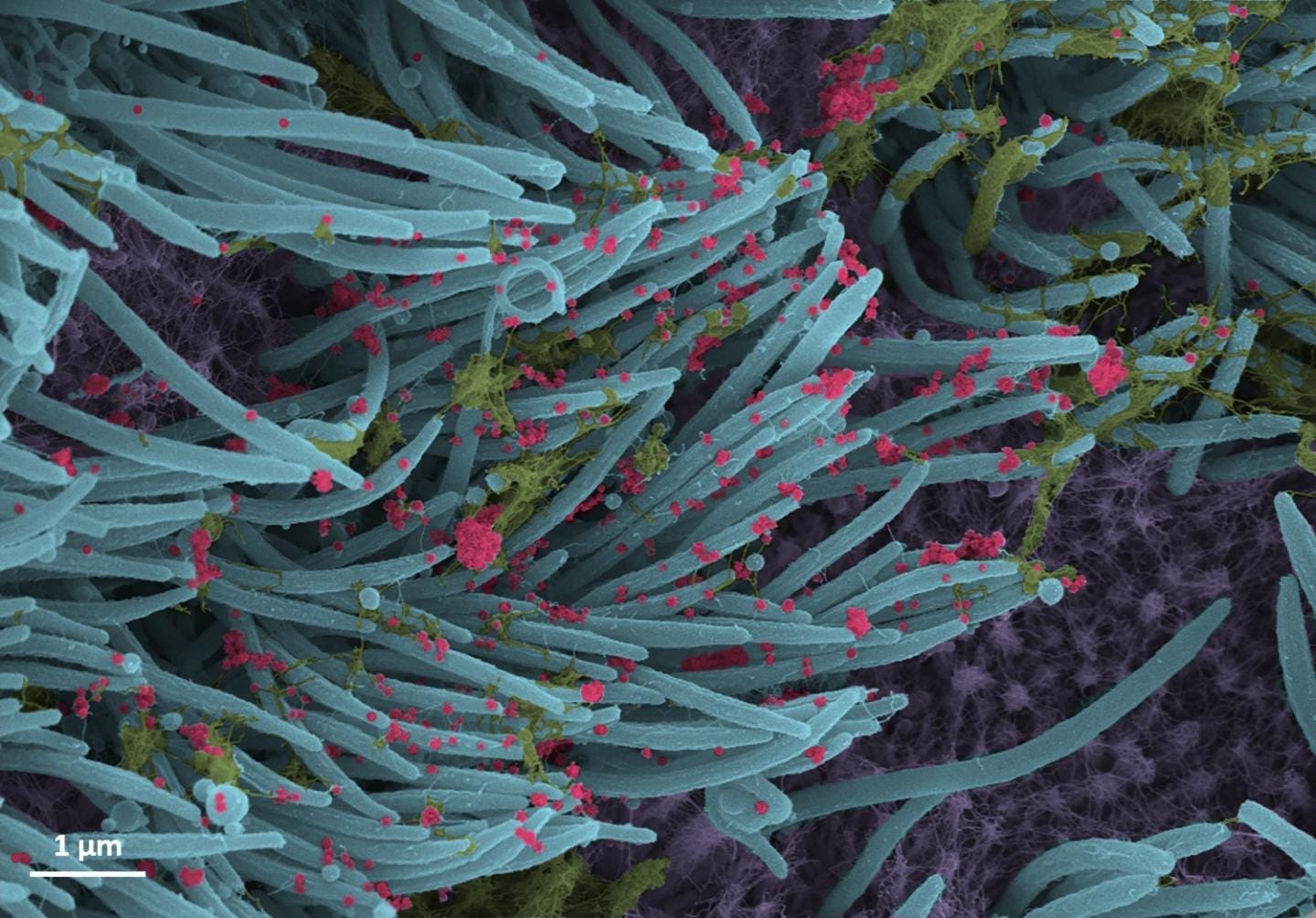
One solution is to use broad-spectrum antiviral prophylactics rather than treatments. Prophylactics, similar to vaccines, are taken before we get sick, so they slow viral growth during the early “viral phase” of disease. They should therefore be much more effective than antiviral treatments that are given after the onset of symptoms.
One computational model, published in 2020, suggested that antivirals given prior to SARS-CoV-2 infection could reduce peak viral load by more than a million times compared to antivirals given three days post-symptoms. Unfortunately, converting existing broad-spectrum antiviral treatments to prophylactics just by changing when they’re administered isn’t always possible. Remdesivir is given through intravenous injections, and half of its molecules are cleared from the bloodstream every day.2 Administering remdesivir prophylactically would require constant visits to the clinic for up to 2-hour-long infusions—hardly practical for a healthy person.
New broad-spectrum antiviral prophylactics could overcome these practical challenges by being more easily delivered, longer-lasting, or both. Some promising approaches build on the conceptual underpinnings of porcine gastric mucins and DRACOs/dsCAREs—that is, having broad-spectrum antiviral activity by being a physical, mucus-like barrier or by targeting double-stranded RNA—but with specific design choices to enable prophylactic use.
Last year, a research team at Harvard University reported the development of “PCANS,” a broad-spectrum antiviral prophylactic nasal spray designed to be used daily. Like mucus, PCANS forms a physical barrier in the nasal cavity that traps incoming virus particles. In mice, at least, the nasal spray was safe and effective; PCANS-treated mice survived influenza infections, whereas control mice died. In a separate study, mice dosed with PCANS daily for two weeks had no significant side effects, and the spray lingered in their nasal cavities for eight hours after each dose.
A similar prophylactic, “SHIELD,” is made of polymer-based microparticles. The microparticles are inhaled as a dry powder which, upon contact with mucus in the lungs, swells and forms a hydrogel network that reinforces the native mucus layer. When inhaled eight hours before infection by different variants of SARS-CoV-2, SHIELD reduced the amount of viral RNA in monkeys by about 50 times in the lungs and 300 times in the nose. Safety tests also showed no toxicity when mice were treated with SHIELD daily for two weeks.
Other approaches could allow for less frequent dosing. DNA delivered to the nose or lungs can express an encoded protein for months to over a year in mice if the vector—the “backbone” of DNA-based medicines controlling the level and timing of expression of the encoded protein—is specifically engineered to be long-lasting. Intranasal or inhalable DNA encoding a broad-spectrum antiviral protein could theoretically make for a prophylactic with once-seasonally or once-yearly dosing. DNA-encoded antiviral proteins delivered to the nose or lungs of mice have previously been shown to protect against influenza or COVID-19. In those experiments, however, the animals were dosed 1-2 weeks before infection with a virus; a better way to test the limits of prophylaxis would be to dose the mice months or even a year before infection.
Our goal at Panoplia is to make antiviral prophylactics that provide months-long protection against pathogens. We’re encoding double-stranded RNA-targeting proteins in long-lasting DNA vectors, and then testing whether our candidates reduce the replication of various viruses in cells over time. Next, we’ll assess whether our DNA vectors protect animals from multiple respiratory viruses for months on end, before ultimately moving them into the clinic.
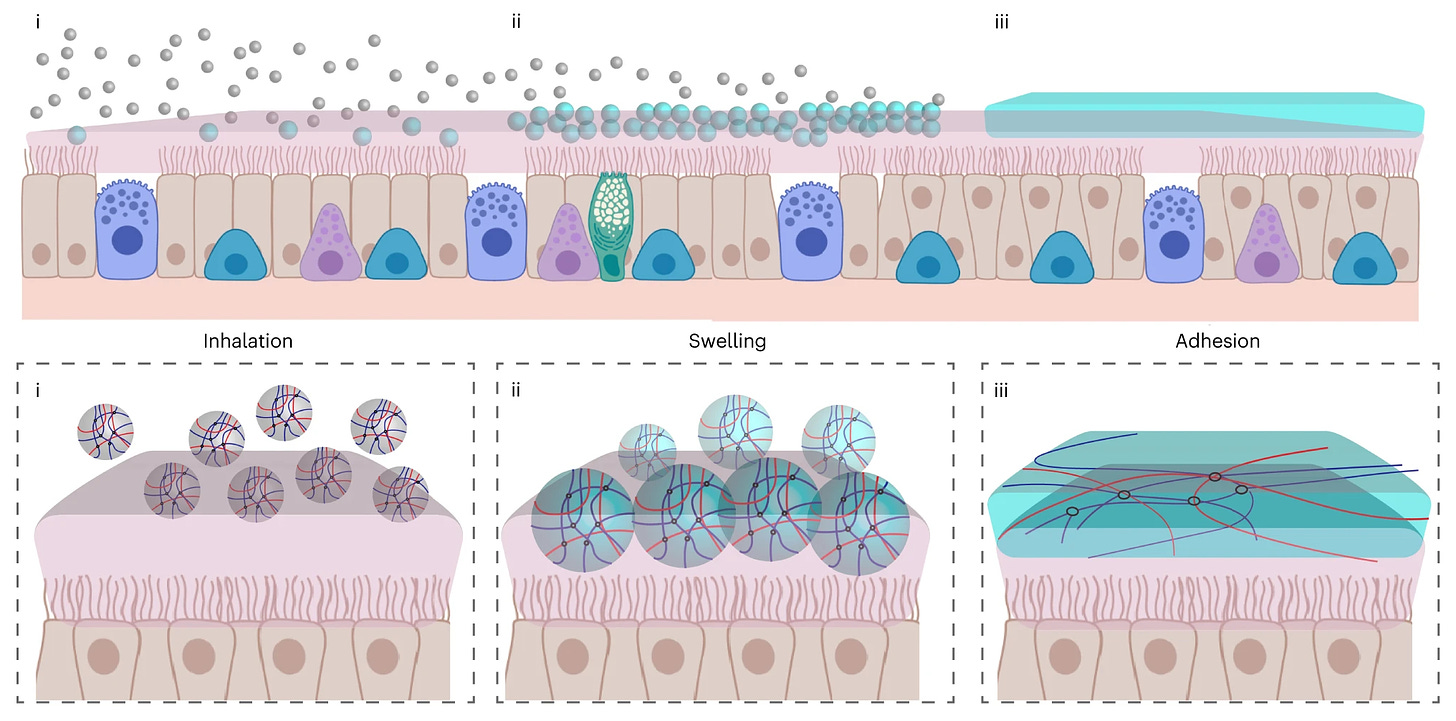
This goal is lofty and may require that we go well beyond existing research. We may need to engineer new double-stranded RNA-targeting proteins, for example, that react against viral double-stranded RNA without overreacting to the small amount of double-stranded RNA present in human cells—a potentially narrow tightrope to walk if this selectivity needs to be maintained for months. We may also need to modify existing DNA vectors so that they express our double-stranded RNA-targeting proteins at optimal levels, and only when they’re needed during infection.
Just as evolutionary experimentation was necessary to shape our innate immune system, we’ll only find out through lab testing.
Boosting Supplies
Making a better broad-spectrum antiviral isn’t enough to prevent the next pandemic. It must also be delivered widely to allow for immediate treatment of patients.
This has proven difficult in the past. At the beginning of the COVID-19 pandemic, the worldwide supply of remdesivir was limited to 5,000 treatment courses. It took Gilead nine months to scale up that number to 500,000. In contrast, hydroxychloroquine—already widely-prescribed for rheumatoid arthritis, lupus, and malaria—reached about 500,000 off-label prescriptions for COVID-19 in just a few weeks.
Besides the fact that remdesivir was at least somewhat effective while hydroxychloroquine proved a false hope, the difference between these two drugs is stark. Whereas hydroxychloroquine had already been approved for diseases with established markets, remdesivir lacked approval for any commercially-relevant indication prior to the pandemic. Without a pre-existing market to provide a steady stream of revenue, there was little incentive for Gilead to manufacture remdesivir in large quantities before COVID-19.
Broad-spectrum antiviral drugs often have trouble finding pre-existing markets because regulators and consumers favor drugs that treat specific diseases, rather than drugs that have broad applicability. When regulators review a new drug application, they weigh the benefits and risks of the drug for a specific disease in a particular patient population. Efficacy against other diseases is not usually considered relevant.
To get their products onto the shelves, then, some broad-spectrum antiviral developers are finding creative ways around these commercial and regulatory challenges. One approach is to avoid regulatory approval entirely. PCANS, for example, is made entirely from components listed on the FDA’s Inactive Ingredients Database and Generally Recognized as Safe list, meaning that its components do not raise safety concerns. As a result, it is classified as a “drug-free” product and does not require FDA approval. PCANS can be freely purchased online.
Similarly, one can repurpose existing drugs as broad-spectrum antivirals if they are determined to be effective as such. Researchers at Yale University recently discovered that neomycin—a common antibiotic present in Neosporin, an over-the-counter topical ointment—stimulates innate immunity and has broad-spectrum antiviral activity. Administering neomycin into the noses of mice protected them from both influenza and SARS-CoV-2 infection. Human volunteers who swabbed their noses with Neosporin twice daily for one week also had stimulated innate immune systems and tolerated the drug well. Since Neosporin is already widely available as an over-the-counter medication, it could be a cheap and easy way to block the spread of future pathogens.
There are downsides to these approaches, of course. Companies may lack incentives to conduct human clinical trials for drug-free antivirals or repurposed drugs. Drug-free antivirals can enter the market without such trials, while repurposed drugs are often off-patent, which makes clinical trials a high-cost, low-reward proposition for sponsors. And without human clinical trials, we can’t be certain that broad-spectrum antivirals developed in these ways are truly effective. As a result, there remains significant value in navigating traditional regulatory paths for a new broad-spectrum antiviral.
At Panoplia, we are making broad-spectrum antivirals that will require approval by regulatory authorities before entering the market. Our strategy involves identifying market niches underserved by existing antivirals and vaccines, such as for immunocompromised patients who currently lack effective prophylactic options for respiratory infections due to reduced vaccine efficacy in this population. Given the scarcity of alternatives, our antivirals could potentially be best-in-class for this patient population, even for a specific virus.
However, this objective comes with a big caveat: the market and the competition are ever-changing. Other research teams have been developing virus-specific prophylactics against respiratory viruses like COVID-19 and influenza for immunocompromised patients; if they are successful, it would be harder for our broad-spectrum antivirals to reach the market for these specific use cases. We expect that we’ll have to re-assess the specific disease indication and patient population on an ongoing basis, especially as we enter into conversations with partners who would help run the clinical trials. Only the end goal remains constant: finding an existing market niche that can help bring our antivirals to the shelves in non-pandemic times.
All in all, creating a day zero antiviral won’t be easy. It’ll require us to re-invent our concept of what broad-spectrum antivirals look like: not only viral protein-targeting treatments like remdesivir, but also fundamental property-targeting prophylactics like PCANS, SHIELD, and DNA-encoded DRACOs/dsCAREs. And while the inexorable force of evolution shaped the tools of our innate immune system against viruses over millions of years, we won’t be able to rely on similar pressures or timelines to forge our day zero antivirals.
The good news is that the innate immune system provides us with a blueprint. We know that we should make antivirals that target the most fundamental properties of viruses, are prophylactic, and are widespread. The wheels of innovation are already in motion. The question is whether the protections we discover will be widely available in time for the next pandemic.
Brian Wang is co-founder and Executive Director of Panoplia Laboratories, a nonprofit developing broad-spectrum antivirals using synthetic biology. You can reach him at @bscwang on Twitter or at brian.wang@panoplialabs.org by email.
Cite: Brian Wang. “Day Zero Antivirals for Future Pandemics.” Asimov Press (2024). DOI: https://doi.org/10.62211/72pr-26gf
Technically, remdesivir is converted into an active metabolite in just a few hours in the bloodstream; it is this metabolite, “GS‐441524,” which has a half-life of one day.
Nice article Brian!
> Companies may lack incentives to conduct human clinical trials for drug-free antivirals or repurposed drugs. Drug-free antivirals can enter the market without such trials, while repurposed drugs are often off-patent, which makes clinical trials a high-cost, low-reward proposition for sponsors. And without human clinical trials, we can’t be certain that broad-spectrum antivirals developed in these ways are truly effective. As a result, there remains significant value in navigating traditional regulatory paths for a new broad-spectrum antiviral.
Regarding this point - I agree that there will be low incentives for conducting clinical trials for drug-free antivirals or repurposed off-patent drugs, yet I wonder if something similar to an advance market commitment could be used here. In this case, instead of committing to purchase an approved drug, a public funder could instead commit to running a large clinical trial if a company commercialises an antiviral product that does not require regulatory approval and meets some initial criteria (e.g. a pre-specified efficiency in animals or a pilot-clinical trial). This 'advance research commitment' could incentivise innovation for broad-spectrum antivirals outside traditional regulatory pathways, the company benefits as a successful trial will provide the product with credibility and increase market demand, the public benefits as the antiviral can be sold at a lower price than if the company has to recover the cost of phase 3 clinical trial.
The of idea of public funders paying for clinical trials of pharmaceutical products is from the Chapters 9 and 10 of Against Intellectual Monopoly (free download at http://www.dklevine.com/general/intellectual/againstnew.html), where they argue that the public would benefit from reduced patent protection on drugs, and the monopoly pricing afforded by patent protection wouldn't actually be necessary if the Phase 2 and 3 clinical trials required for regulatory approval were publicly funded. The rational is that clinical trial result submitted to regulators are a public good, and should be paid for directly by the public rather than paid for by the pharmaceutical industry (and then recovered in high product prices).
How does your approach compare to that of Kimer Med in New Zealand?