Microscopic traffic: Engineered protein motors walk on DNA
A new study could help build rail networks in living cells.
Mini Motors
Imagine taking a bird’s-eye view of a complex rail network. At each junction, high-speed trains are sorted to the correct track. Passengers are ferried to destinations. Materials move to manufacturing centers; products to consumers. A study by Ibusuki et al., published last week in Science, could take researchers one step closer to building similar systems at the microscopic scale.
For the new study, researchers at the University of Hyogo and the National Institute of Information and Communications Technology of Japan modified a motor protein called dynein, which transports cargo in cells along cytoskeletal fibers known as microtubules. The wide variety of possible cargoes makes dynein an important player in many cellular processes, from cell division to mRNA localization and cell death. However, these microtubule networks are complex and ever-shifting, which makes them difficult to use as reliable tracks for synthetic applications.
DNA, on the contrary, seems perfect for the job. Besides being an information carrier, DNA is also a malleable material that can be programmed to self-assemble into arbitrary 3D structures, a technique known as DNA origami. Ibusuki et al. replaced the part of dynein that binds to microtubules with DNA-recognizing modules from proteins that naturally attach to DNA, such as transcription factors. These engineered motors bind DNA tracks and move in a single direction along them.
The researchers highlight the potential of their approach by designing tracks of linear DNA nanotubes and joining them in Y-shaped junctions. Synthetic dyneins walk on these tracks in a programmable fashion. For example, the choice of DNA sequence, used to build the track, determines the direction that a dynein walks, as well as the path the motors take at a given junction.
Previous examples of synthetic DNA-walkers were either slow or moved in ways that destroyed the tracks they walked on. Ibusuki’s walkers move at speeds close to those of some molecular motors in nature, such as dyneins and kinesins that carry cytoskeletal proteins along axons in the brain. The engineered walkers also leave their tracks intact.
In the future, DNA-walkers like these could be used to swiftly transport DNA-nanostructures within cells, or could be used as vehicles to carry monomers to polymerization centers.
A lot of research, today, aims to understand unique features of DNA that can be exploited to build biological computers capable of storing and processing information, much like conventional electronics do. In the new study, the authors speculate that locomotion on DNA tracks could be smoothly integrated with such DNA computing systems, paving the way for microscopic robots that interact with their environment and perform mechanical tasks.
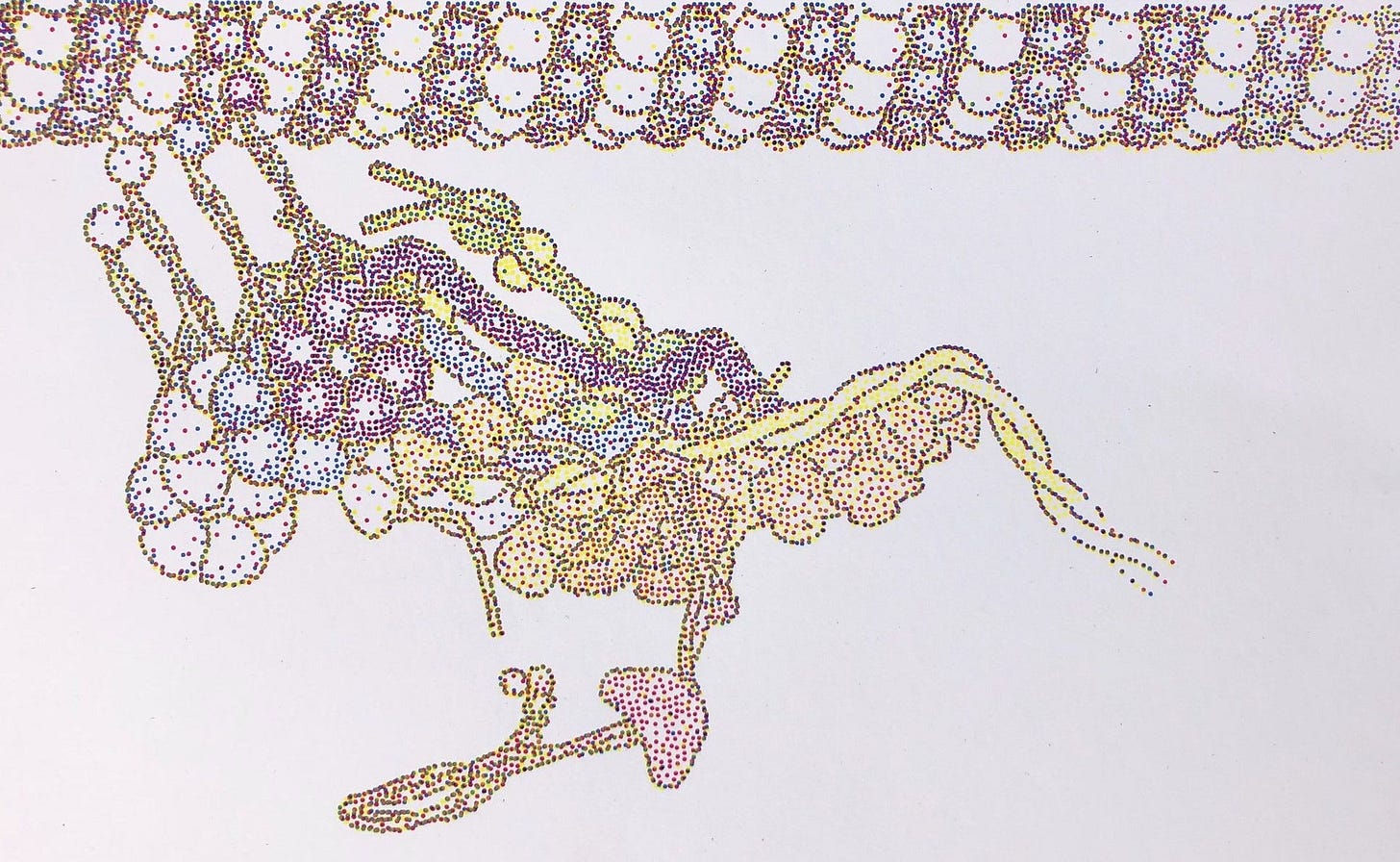
Right feet for the right track
Dynein is made of different functional modules, called domains, and this modular structure makes it a good candidate for protein engineering. One of dynein's domains generates force, while another domain binds to microtubule tracks. For the new study, Ibusuki et al. removed the microtubule-binding domain in dyneins taken from human cells, and replaced it with a set of different, DNA-binding domains from other proteins. Importantly, they focused on domains that recognize non-palindromic sequences — stretches of double-stranded DNA for which the sequence on one strand is different from the sequence on its complementary strand. This allowed the researchers to define the direction that the engineered dyneins travel, simply by changing the orientation of the DNA sequences they recognize.
The DNA ’tracks’ were built from parallel double helices, woven together into a cylinder shape roughly 10 nanometers in diameter. To assess whether the engineered motors could bind DNA and exert force, Ibusuki et al. used something called a gliding assay; basically, they attached the dynein motor domain to a glass surface and left the DNA-binding domain free. Then, they added a solution containing DNA nanotubes. Eleven out of nineteen of the engineered dyneins catalyzed directed gliding of DNA nanotubes, each with a different velocity.
Loading the cargo
Natural cytoplasmic dynein is one of the largest protein complexes in the cell. It is a homodimer —a protein composed of two identical subunits— that requires the binding of co-factors and adaptor proteins to load cargo and walk on microtubules. When cargo is absent, its two identical motor domains become fused, preventing dynein from binding its tracks. This feature is thought to prevent free motors from blocking microtubule traffic in cells. To prevent this self-inhibition, Ibusuki et al. separated the motor domains of their synthetic dyneins by placing a rigid rod domain between them.
The synthetic dimers were able to walk along nanotubes without any cargo, but only for a distance of about 700 nanometers. However, this distance improved when several motors were collectively carrying the same cargo. In cells, adaptor proteins naturally load cargo onto dyneins; for their synthetic counterparts, the researchers again turned to DNA origami to create an appropriate load. They designed DNA scaffolds with chemical anchors to which the motors could be attached (using a so-called SNAP-tag), thus creating cooperative complexes in which fifteen motors could bind to, and carry, the same DNA scaffold cargo. The complexes walked along 7 micrometers of DNA, at a velocity of 24 nanometers per second, with their cargo in tow. There is certainly strength in numbers.
Taming traffic
Driving along an endlessly straight highway might take you a long way, but to build an actual road network you will certainly need junctions. Accordingly, Ibusuki et al.assembled Y-junctions composed of three nanotube strands joined by a central knot. By choosing the orientation of the motor binding sites on each of the strands, the DNA-walkers could be programmed to move towards the junction or away from it.
Finally, Ibusuki et al. designed junctions that can sort different types of motors. For this, they used two of their synthetic dyneins, respectively fused to the DNA-binding domain of the human transcription factor LEF1 or the σE4 sigma factor from E. coli. The pair was chosen because of the absence of cross-talk and because they walk at similar speeds. By assembling a nanotube that contains recognition sequences for both types of motors, the researchers achieved the equivalent of a street with two specialized lanes.
With these tools, Ibusuki et al. designed four types of junctions. When only a single kind of dynein is involved, dispersers divert traffic that reaches the junction through an incoming track into any of two outgoing ones. On the contrary, an aggregator junction combines two incoming tracks in a common outgoing one. In the more complex case of two types of dynein being present, sorter and integrator junctions perform analogous operations. They either separate incoming dyneins into opposite, specialized tracks according to their type, or they merge traffic from individual, specialized tracks into an outgoing track shared by both types.
Single junctions don’t make a network, but it is easy to see how this approach could be scaled and adapted to implement more complex, cargo-delivering programs. And who knows? Maybe, one day, someone will actually build a microscopic system that mimics the rail yards and highways that keep our economies running.
By Joaquín Gutiérrez
About the Author
A physicist turned synthetic biologist, Joaquín Gutiérrez works as a PhD student at ETH Zurich developing methods to control bacterial communities. His interests include genetic circuits, microbial ecology, evolution and discovering new interests. In his free time, he writes poetry and uses a piano to study his neighbor's stress response.
Reach him at joaquin.gutierrez@bsse.ethz.ch or on Twitter @GutierrezJoa