A couple of miles south of the University of Illinois is an area roughly the size of Central Park. Corn, soybeans, and cowpeas grow in abundance. Though it looks much the same as the surrounding fields, this is not just another farm: it’s the oldest continuously run agricultural research site in the U.S. And those plants? They’ve been engineered to grow bigger and faster, their DNA modified to speed up one of life’s most important chemical reactions: photosynthesis.
Plants use photosynthesis to harness energy from the sun, pulling carbon dioxide out of the air and turning it into sugars. Some plants are much better at this than others; the more sugar a plant makes, the bigger it grows.
This growth variability between plants is now a target of opportunity for crop scientists, who are searching for new ways to boost productivity. Their work is urgent. Food production will need to roughly double to fully feed ten billion people, the world’s expected population by 2050.
Conventional yield-boosting techniques, such as pesticides, drought resistance, and better fertilizers have increased crop yields by less than two percent per year. This modest rate of improvement is expected to slow as the earth warms, especially in tropical regions hit by droughts or flooding. Our best hope to boost crop yields is to tinker with photosynthesis directly, either by accelerating photosynthesis enzymes or by doing away with photosynthesis entirely and giving plants a more efficient alternative.
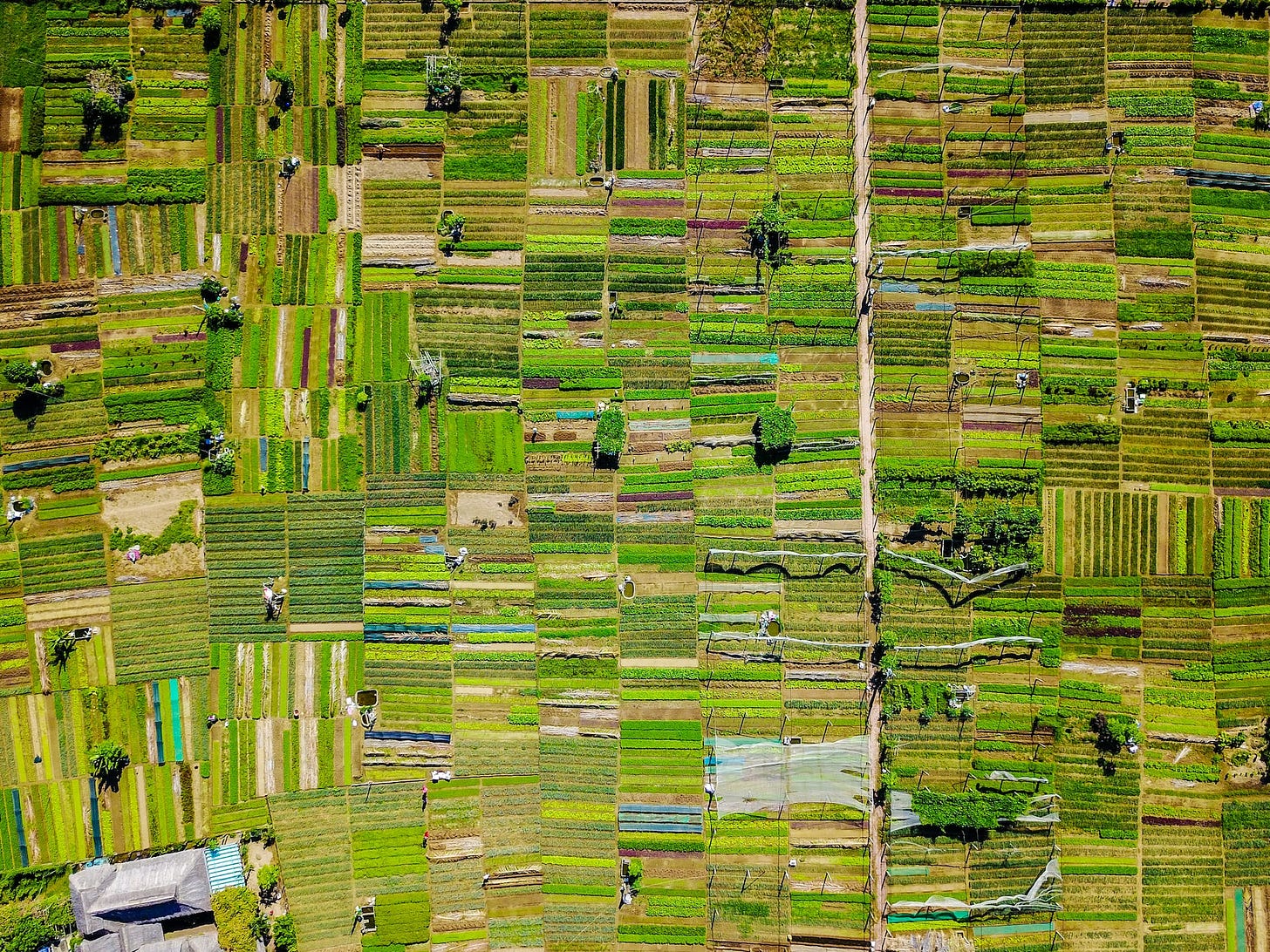
A Better Rubisco
At the University of Illinois’ South Farm, researchers from the RIPE project (Realizing Increased Photosynthetic Efficiency) are working on ways to amp up photosynthesis. They have already built plants that carry a modified form of Rubisco, a key photosynthesis enzyme that evolved 3.5 billion years ago, when carbon dioxide in the atmosphere was much higher.
Rubisco works slowly in today’s relatively low-carbon environment because it often mistakes oxygen for CO2. The enzyme captures just three molecules of carbon dioxide per second. Enzymes that break down food in our stomachs, by contrast, carry out hundreds of reactions per second.
Rubisco’s size, complexity, and unique evolutionary history make it difficult to manipulate. But a few years ago, plant engineers at Cornell University took a “fast” Rubisco from algae, loaded it into tobacco plants, and found that the plants’ photosynthesis sped up by nearly 50%. The RIPE team is using similar strategies to make bigger food crops.
When RIPE boosted photosynthesis in soybeans, “we found that the quality of the grain and the amount of protein is unchanged, but we get 20% more food,” says Stephen Long, a plant biologist and the director of RIPE, whose $45 million budget is partly funded by the Bill and Melinda Gates Foundation.
RIPE’s next target is cowpea, a legume whose best-known cultivar is black-eyed pea. Cowpea is a staple crop in food insecure regions in Sub-Saharan Africa. About 30% of Africans suffer from chronic hunger and malnutrition, according to the Food and Agriculture Organization, and that number is increasing, says Long. Hot days in the region are becoming more frequent, which hurts crop yields. Cowpea is a leading source of protein for over 200 million people, but Nigerian farmers, its main producers, cannot grow enough to meet demands.
Unfortunately, boosting cowpea and rice yields by modifying Rubisco directly will take a really long time. For a single experiment, it used to take three months to modify the enzyme, insert it into plants, and wait for them to grow, according to Maureen Hanson, a plant geneticist at Cornell. That slow pace handicapped experiments because researchers can only test a few versions of engineered Rubisco each year.
Hanson published a study in late 2020 that could sharply cut that time. She took Rubisco from a plant and placed it inside of E. coli bacteria, which double in population every thirty minutes or so. “Now we can say, ‘Oh, let’s change that amino acid there,’ and see what happens” in a few days, rather than months, she says.
Other scientists are less concerned with engineering plant Rubiscos because they think that a better version of the enzyme is already hiding in nature — no engineering required.
Israeli molecular biologist, Ron Milo, searched genetic databases and found 33,000 different types of Rubiscos in thousands of plant and microbial species. His team at the Weizmann Institute of Science studied a subset of them in test tubes and watched to see which ones worked fastest. Milo called the experiment “the Rubisco-lympics.”
Back in June 2020, his team found a Rubisco from cyanobacteria, a photosynthetic organism, that worked six times faster than a typical plant version. Milo’s team is now inserting those enzymes into crops to see whether it makes them larger.
Other researchers, though, see Rubisco as a dead end entirely. “Rubisco has evolved over billions of years,” says German bioengineer Tobias Erb of the Max Planck Institute for Terrestrial Microbiology. “You don't teach this old enzyme new tricks.”
Go Synthetic
In Asia, a hectare of land today feeds 27 people. By 2050, that same land will need to feed 43 people. Traditional rice breeding has only increased yields by about 1% year-over-year.
The C4 Rice Project — a global consortium of researchers from 7 institutions in 5 countries — aims to boost yields by converting rice’s photosynthesis from its native C3 to another type, called C4.
Most C4 plants (like maize) are much more efficient because they use two separate compartments for photosynthesis. As CO2 comes into the cell, it is first fixed by pep carboxylase, an enzyme that doesn’t inadvertently bind to oxygen. The product of this reaction is then shuttled to a different cell, where Rubisco lies in wait. C4 plants are basically CO2 concentrators — less oxygen gets to Rubisco, and photosynthesis is faster as a result.
Back in October 2020, the C4 team took five genes from maize and ported them into rice. The added genes didn’t boost crop yields much, but it was an early step that indicated a C3 to C4 conversion might be possible.
And just a few days ago, scientists discovered that Portulaca plants perform C4 and Crassulacean acid metabolism (CAM, another carbon fixation pathway) photosynthesis in the same cell, thus providing evidence that the hard part of the C4 Rice project — namely, spatially isolating the different steps in photosynthesis — might not be needed after all.
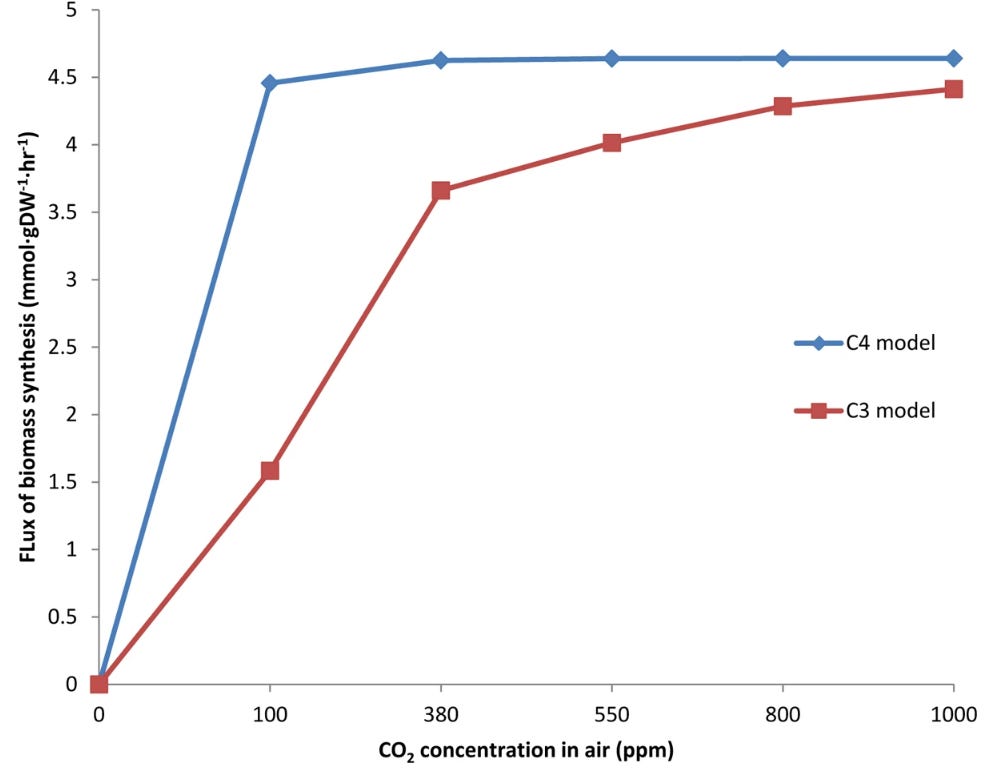
If RIPE and the C4 Rice projects fail (and they might), other researchers will be ready with alternatives.
In the past year, both Milo and Erb have entirely rebuilt the chemical process for photosynthesis in their labs. In 2016, Erb created an artificial photosynthesis system, called the CETCH cycle, using 17 different enzymes from various plants and microbes, including three enzymes that his research team designed from scratch. Each “designer” enzyme was 3D-modelled on a computer, created in the laboratory, and tweaked over a four-year period before the system worked as expected.
When the enzymes were added to a test tube, the artificial photosynthesis was about five times faster than natural versions. But Erb, at the time, couldn’t get the enzymes to work in living cells: “It's like building an engine and then trying to put that into an existing car,” he says.
A couple years ago, in Science, Erb’s group also reported an artificial chloroplast — made from spinach chloroplast membranes and their CETCH enzymes — that uses sunlight to convert CO2 to sugar. And last year, Erb’s team built a rationally-engineered enzyme that rivals the efficiency of natural CO2-fixing enzymes. Based on computer modeling, they predict that the enzyme could boost the efficiency of synthetic CO2 fixation by 150%.
Future Nightmares
Erb told me, last year, that he plans to add some of his artificial photosynthesis enzymes to plants, provided that he can get European approval (which seems unlikely). But he was largely moot on details.
Cornell’s Hanson applauds Erb’s work but is skeptical that artificial photosynthesis would be widely adopted.
“Whoever commercializes these plants — probably one of the large plant-breeding or seed companies — would have to go through a long regulatory process to get approval,” says Hanson. “And that can take a really long time, unfortunately.” In contrast, Hanson thinks that plants with a modified Rubisco could be rolled out to farms in the next five years because only one gene would have to be altered.
The cost to test engineered plants, says Stuart Smyth, an agricultural biotechnologist at the University of Saskatchewan, is hard to predict ahead of time, but can stretch into the hundreds of millions of dollars.
Still, the crops would likely be approved in the U.S., predicts Jennifer Kuzma, a biotechnology policy expert at North Carolina State University. That’s because, when the U.S. Department of Agriculture evaluates an engineered crop, says Kuzma, it only checks to see if the modified genes make the plants more likely to be eaten by beetles or other pests.
“That’s a narrow scope of risk,” she says. “I cannot think of a way that increasing photosynthesis would increase plant pest risk.”
Critics of genetically modified crops say that there are ways to feed the planet without boosting photosynthesis. For example, cereal harvests (think wheat, rice, and corn) have boomed in recent decades, with yields increasing at about twice the rate as the human population, according to The World Bank, despite being grown on the same amount of land for the last fifty years.
Those yields could be boosted further by switching from annual to perennial grains, which grow for more than one season before being harvested, says Timothy Crews, a soil ecologist at The Land Institute. He acknowledges, however, that much more breeding and research will be needed to see major yield improvements. There are huge structural obstacles to switching to perennials, too: Over 90 percent of U.S. corn, cotton, and soybeans are genetically engineered. These seeds are monopolized by agricultural companies whose sales depend on annual varieties.
Feeding a growing population is not a simple feat, but clearing more land for agriculture, or planting more crops, is certainly not the answer. Forests are already being decimated to make way for farms in Brazil and elsewhere. Instead of expanding land, we need to bolster yields.
RIPE’s Long thinks that every approach — new Rubiscos, artificial photosynthesis, better fertilizers, perennial crops — are worth exploring. And though he’s confident that biotechnology can eventually meet global food demand, he’s “a lot less optimistic that opposition to GM crops and the complex regulatory framework that this has created will allow it by 2050.”
Improving crop yields will demand that scientists, politicos, and farmers work together, Long says.
“I don’t think we can say that one approach is the solution to everything.”
Last weekend, I spoke with Tessa Alexanian, the Safety & Security Program Officer at iGEM. “Whatever happened to the C4 Rice project?” we wondered. The conversation reminded me of this unpublished article, hidden on my computer’s hard drive, about some of the promising ways in which scientists are engineering photosynthesis. This took a long time to report and write, and I’ve added some recent research advances — thanks for reading!
Awesome article! However, I would like to note that Ron Milo and co-authors work was only able to computationally analyze the ~33k Rubisco homologs. They were not able to test all of the retrieved homologs ( ~33k) in a test tube; however, they did purify and experimentally test ~140 sequences.
Overall, I really enjoyed the article and thanks for sharing it!
First hyperlink under "Future Nightmares" isn't working.
Fascinating read. I strongly dislike pesticides (like glyphosate) as a consumer wary of pollutants that contaminate food like bioaccumulation of PFAS. To most researchers, I acknowledge that I seem exceedingly cautious. Is it accurate to say that concerns over pesticide use should have no bearing on attitudes over "hacking photosynthesis?" It seems like the advantage here is being able to increase biomass yield without resorting to slathering mutagens on crops. Although, I do reserve slight concern over the implications of the innovations discussed here on nutritional yield (e.g do the new plants really have the same amount of protein/other nutrients; do the new modifications not impact how plants absorb nutrients in any significant way?)