Bloodborne CRISPR Treats Genetic Disease - 2021.06.28
Plus: Other research and news in biotechnology.
☀️ Good morning.
Inspect every piece of pseudoscience and you will find a security blanket, a thumb to suck, a skirt to hold. What does the scientist have to offer in exchange? Uncertainty! Insecurity!
—Isaac Asimov
Were you forwarded this email? You can subscribe here.
Phase I CRISPR Blood Trial
A first-of-kind Phase I clinical trial has treated six patients with a hereditary condition, called transthyretin amyloidosis, using CRISPR injected into the bloodstream. The findings were reported in The New England Journal of Medicine. The trial was funded by Intellia Therapeutics and Regeneron Pharmaceuticals.
Read additional news coverage at Science Magazine.
How It Works: Transthyretin amyloidosis is a progressive, fatal disease. Patients with the condition slowly accumulate amyloid fibrils, formed from a misfolded protein, in tissues around their body. The condition is caused by mutations in the transthyretin gene, TTR. Most treatments for the condition use chemicals to stabilize the misfolded proteins and slow down their accumulation in tissues.
For this phase I clinical trial, the researchers tested a CRISPR-Cas9 therapy in six patients with a hereditary form of the condition. They called their medicine NTLA-2001. Previous experiments in nonhuman primates and mice led to a long-term reduction (~95%) of the misfolded TTR protein in blood serum.
The CRISPR-Cas9 system, in this Phase I trial, was delivered intravenously (into the bloodstream). The researchers used a single guide RNA targeting the human TTR gene, as well as a codon-optimized Cas9 protein from S. pyogenes. They packaged both the sgRNA and the Cas9 protein into a “proprietary lipid nanoparticle,” which carries them through the bloodstream to the liver.
The researchers tested two doses of the lipid particles. Three patients received a dose of 0.1 mg per kilogram body weight, and three patients received a dose of 0.3 mg per kilogram. The genetic therapy was effective, and the study “showed durable knockout of TTR after a single dose,” according to the authors. The researchers assessed each study participant after 28 days.
In the ‘low dose’ group, participants had a 52% reduction in serum TTR levels compared to baseline. In the ‘high dose’ group, participants had a 87% reduction in serum TTR levels compared to baseline.
Why It Matters: This is the first CRISPR clinical trial to be delivered straight into the bloodstream of people. It is a phase I clinical trial, so larger trials for safety and efficacy will need to be performed. Still, it is a promising genetic treatment for this rare, inherited condition.
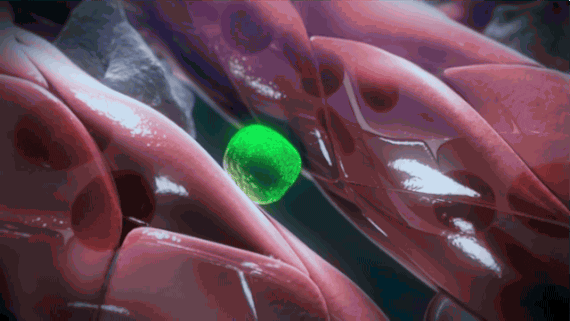
Tunable RNA Lifetimes
A new collection of “modular degradation-tuning RNAs,” or dtRNAs for short, can adjust the lifetime of RNA sequences. Tuning the stability of RNAs could open up new possibilities for researchers building genetic circuits inside of living cells. A study describing the dtRNAs appeared in Nature Chemical Biology and was led by researchers from Arizona State University and Boston University.
How It Works: The E. coli ompA gene encodes a circular protein that sits in the outer membrane of cells and allows small molecules to enter. The 5’ untranslated region of this gene forms a secondary structure that changes the stability of RNA sequences.
The researchers noticed that, by modifying the ompA 5’ sequence and appending it to the start of other genes, they could tune the lifetime of other RNA sequences. And by tuning the lifetime of RNA sequences, they could change the amount of protein that gets made.
They made and characterized more than 80 versions of these dtRNAs, each of which can tune gene expression (via changing RNA stability) to a varying degree. The dtRNAs “enable modulation of transcript stability over a 40-fold dynamic range in Escherichia coli,” according to the authors.
Each dtRNA forms a stem-loop structure; different stem lengths and “loop” sizes change the stability of the RNAs in predictable ways. Higher GC content in the stem, shorter stem lengths, and smaller loops were all associated with higher RNA stability and longer RNA lifetimes.
In a series of experiments, the researchers used these dtRNAs to tune the dynamics of genetic circuits, to build paper-based viral diagnostics, and to regulate gene expression in living E. coli cells.
Why It Matters: The authors have made a large collection of well-characterized, “degradation-tuning” RNAs that can be used to reliably control the stability of arbitrary RNA sequences. These dtRNAs will be useful for optimizing genetic circuits and are a nice addition to the synthetic biology toolbox.
Hypermutations Make Strong Antibodies
Yeast engineered with a ‘super fast mutation’ system can make strong antibodies against SARS-CoV-2, the virus that causes COVID-19, faster than traditional methods. That’s according to a new study in Nature Chemical Biology, led by researchers at the University of California, Irvine and Harvard Medical School.
How It Works: OrthoRep, first reported in 2018, is a system that can be used to mutate genes 100,000-fold faster than normal, genome mutation rates. It works by using an orthogonal DNA polymerase that recognizes a specific plasmid in yeast. The DNA polymerase is “error-prone,” which means it makes a lot of mistakes, and it only copies genes encoded on its corresponding plasmid.
Now, researchers have used OrthoRep to create antibodies against the SARS-CoV-2 S glycoprotein. To do that, they coupled OrthoRep with a yeast surface display system.
First, the researchers placed a gene encoding an antibody on the orthogonal plasmid, and used the error-prone polymerase to mutate it in yeast. In those same cells, the researchers also expressed an adhesion receptor protein, called Aga2p, and a cell wall anchoring protein, called Aga1p. As the antibodies evolve, they link up with these other proteins and are displayed on the yeast surface. The strength of the evolved antibodies can then be assessed using flow cytometry.
The researchers used this system (called AHEAD, or ‘autonomous hypermutation yeast surface display’) to create improved anti-SARS-CoV-2 nanobodies. Several antibodies had more than 100-fold neutralization improvements compared to the wildtype antibodies after only a few cycles through AHEAD.
Why It Matters: This paper presents a rapid technique to evolve potent antibodies against SARS-CoV-2. It could also probably be used to make antibodies against other pathogens or proteins, which makes it a widely useful tool for biotechnology and medicine.
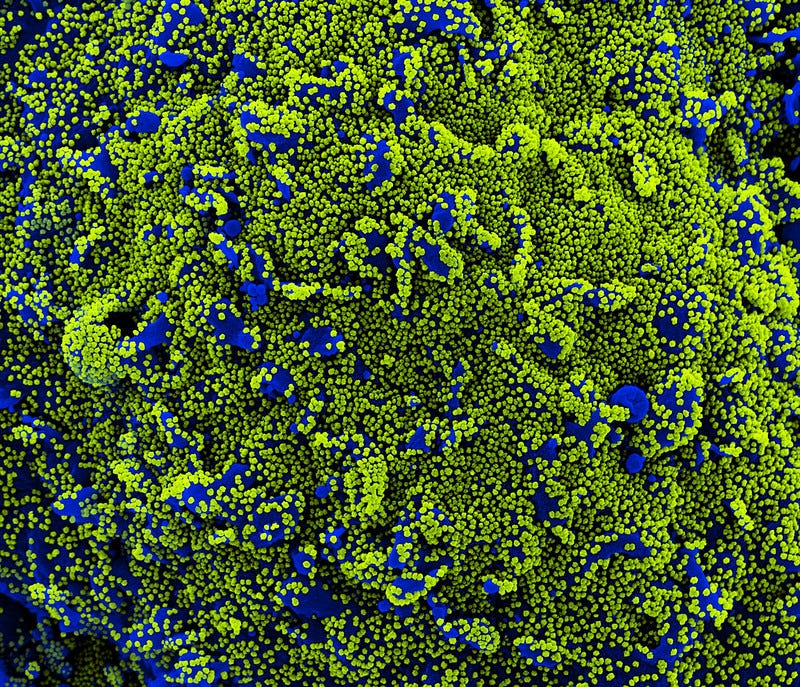
🧫 Other Studies This Week
Am I missing coverage on a certain topic? Leave a comment on this post.
Studies now include abbreviated author names.
Artificial Life
Proton gradients from light-harvesting E. coli control DNA assemblies for synthetic cells. Jahnke K. et al. Nature Communications (Open Access). Link
Chemical-mediated translocation in protocell-based microactuators. Gao N. et al. Nature Chemistry. Link
(Review) Caged lipids for subcellular manipulation. Farley S. et al. Current Opinion in Chemical Biology. Link
Biosensors
A Biosensor for Detection of Indole Metabolites. Wang J. et al. ACS Synthetic Biology. Link
(Review) Engineering bionanoparticles for improved biosensing and bioimaging. Yur D. et al. Current Opinion in Biotechnology. Link
Cell-Free Systems
High-Efficiency Protection of Linear DNA in Cell-Free Extracts from Escherichia coli and Vibrio natriegens. Norouzi M. et al. ACS Synthetic Biology. Link
(Preprint) Point-of-care analyte quantification and digital readout via lysate-based cell-free biosensors interfaced with personal glucose monitors. Zhang Y. et al. bioRxiv (Open Access). Link
Fundamental Discoveries
Cytosine base modifications regulate DNA duplex stability and metabolism. Rausch C. et al. Nucleic Acids Research (Open Access). Link
Gene Drives
A genetically encoded anti-CRISPR protein constrains gene drive spread and prevents population suppression. Taxiarchi C. et al. Nature Communications (Open Access). Link
Genetic Code Expansion
(Preprint) Engineered human induced pluripotent cells enable genetic code expansion in brain organoids. van Husen L. et al. bioRxiv (Open Access). Link
(Preprint) Genetic code expansion in the engineered organism Vmax X2: High yield and exceptional fidelity. Santiago S. et al. bioRxiv (Open Access). Link
Genetic Engineering & Control
Single-component near-infrared optogenetic systems for gene transcription regulation. Kaberniuk A.A. et al. Nature Communications (Open Access). Link
Cytosine and adenosine base editing in human pluripotent stem cells using transient reporters for editing enrichment. Tekel S.J. et al. Nature Protocols. Link
A transferrable and integrative type I-F Cascade for heterologous genome editing and transcription modulation. Xu Z. et al. Nucleic Acids Research (Open Access). Link
Medicine & Diagnostics
Directed evolution of potent neutralizing nanobodies against SARS-CoV-2 using CDR-swapping mutagenesis. Zupancic J.M. et al. Cell Chemical Biology (Open Access). Link
Integrating programmable DNAzymes with electrical readout for rapid and culture-free bacterial detection using a handheld platform. Pandey R. et al. Nature Chemistry. Link
(Preprint) Rational engineering of an erythropoietin fusion protein to treat hypoxia. Lee J. et al. bioRxiv (Open Access). Link
Metabolic Engineering
Improved pyrrolysine biosynthesis through phage assisted non-continuous directed evolution of the complete pathway. Ho J.M.L. et al. Nature Communications (Open Access). Link
(Preprint) Targeting riboswitches with synthetic small RNAs for metabolic engineering. da Costa Ribeiro Lins M.R. et al. bioRxiv (Open Access). Link
Engineered yeast tolerance enables efficient production from toxified lignocellulosic feedstocks. Lam F.H. et al. Science Advances (Open Access). Link
Engineering yeast subcellular compartments for increased production of the lipophilic natural products ginsenosides. Shi Y. et al. Metabolic Engineering. Link
(Review) Mining and unearthing hidden biosynthetic potential. Scherlach K. and Hertweck C. Nature Communications (Open Access). Link
Microbial Communities
Engineering a Bifunctional ComQXPA-PsrfA Quorum-Sensing Circuit for Dynamic Control of Gene Expression in Corynebacterium glutamicum. Liu H. et al. ACS Synthetic Biology. Link
An evolutionary algorithm for designing microbial communities via environmental modification. Pacheco A.R. and Segré D. Journal of the Royal Society Interface (Open Access). Link
Plants
Genome design of hybrid potato. Zhang C. et al. Cell. Link
Protein Engineering
(Preprint) Chimeric GPCRs mimic distinct signaling pathways and modulate microglia responses. Schulz R. et al. bioRxiv (Open Access). Link
(Preprint) Dendrimer-like supramolecular assembly of proteins with a tunable size and valency through stepwise iterative growth. Bae J.H. et al. bioRxiv (Open Access). Link
Systems Biology, Modelling & Quantitative Studies
(Preprint) Yeast cells under glucose-limitation environment need increased response cost for osmostress defense. Luo C. et al. bioRxiv (Open Access). Link
Tools & Technology
Multiplexed bioluminescence-mediated tracking of DNA double-strand break repairs in vitro and in vivo. Chien J.C. et al. Nature Protocols. Link
SBOLCanvas: A Visual Editor for Genetic Designs. Terry L. et al. ACS Synthetic Biology. Link
Light-inducible deformation of mitochondria in live cells. Song Y. et al. Cell Chemical Biology. Link
Genome-wide interrogation of gene functions through base editor screens empowered by barcoded sgRNAs. Xu P. et al. Nature Biotechnology. Link
(Preprint) GeneTargeter: Automated in silico design for genome editing in the malaria parasite, Plasmodium falciparum. Cárdenas P. et al. Research Square (Open Access). Link (Check out the web tool.)
Miscellaneous Topics
A catalog of the diversity and ubiquity of bacterial microcompartments. Sutter M. et al. Nature Communications (Open Access). Link
Sequence Preference and Initiator Promiscuity for De Novo DNA Synthesis by Terminal Deoxynucleotidyl Transferase. Schaudy E. et al. ACS Synthetic Biology (Open Access). Link
(Preprint) UNIGEMS: plasmids and parts to facilitate teaching on assembly, gene expression control and logic in E. coli. Siddall A. et al. bioRxiv (Open Access). Link
(Perspective) Biofoundries are a nucleating hub for industrial translation. Farzaneh T. and Freemont P.S. Synthetic Biology (Open Access). Link
Building Biofoundry India: Challenges and Path Forward. Panda B. and Dhar P.K. Synthetic Biology (Open Access). Link
🖥️ Tweet of the Week
Plant-based materials, with a banana for scale.

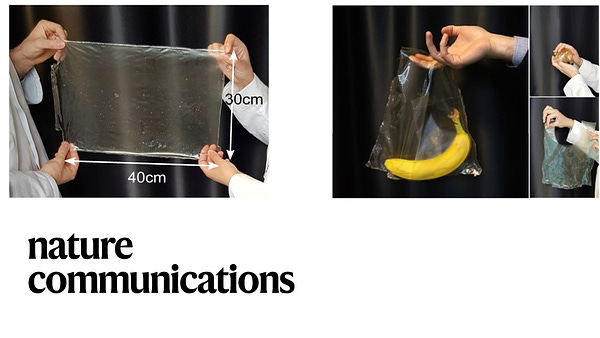
✨ Around the Web
Miscellaneous news.
BACTERIAL BOMB SNIFFERS: E. coli have been engineered to fluoresce when exposed to 2,4-dinitrotoluene, a chemical found in TNT. The engineered cells could be used to hunt down landmines. The New York Times. Link
LAB LEAK PROFILE: Alina Chan, a postdoc at Harvard/MIT’s Broad Institute, was amongst the earliest people to publicly advance the lab-leak theory for SARS-CoV-2. Next, she plans to disappear. MIT Technology Review. Link
TINY FACTORIES: Researchers are engineering B cells to produce proteins for people with enzyme deficiencies. This is a great explainer article. Nature Biotechnology. Link
TREE SUCKERS: A company called Living Carbon is engineering trees that can capture and store more carbon than conventional trees. It’s part of a push towards carbon-negative technologies that can reduce atmospheric greenhouse gases. Fast Company. Link
PSYCH MONEY: Two European psychedelics companies had IPOs this week: atai Life Sciences, from Berlin, and GH Research, of Dublin. Both companies are manufacturing drugs with the intention of treating mental health conditions. Labiotech.eu. Link
ANCIENT CARBS: New clues from Göbekli Tepe, an ancient temple in Turkey, suggest that humans have been growing and using grains far earlier than previously thought. Stone vessels at the site also suggest that these ancient peoples were making beer. Nature. Link
Until next time,
— Niko
Thanks for reading Cell Crunch. If you enjoy this newsletter, please share it with a friend or colleague. You can reach me on Twitter @NikoMcCarty or via email.