A Button, Under the Skin, Releases Insulin from Electro-Sensing Cells
By fusing piezoelectrics and electro-sensitive cells, a "push-button" device produces insulin in a mouse model of Type I diabetes.
Synthetic biologists often say that cells are programmed with genetic circuits, thus drawing an implicit connection between biological engineering and computer science. This analogy fails, in many ways, because it equates the messiness of biology to the consistency of computers. An executed block of code performs the same way every time; the cytoplasm of a cell, by comparison, is jam-packed with RNA and proteins. Stochastic events underlie much of biology, and it is often difficult to make definite, quantitative measurements.
Perhaps this is why, in recent years, I’ve been most excited about the intersection of synthetic biology and electronics, rather than their overarching fusion.
Synthetic biology became more reproducible when robots entered the experimental fray (thanks, Opentrons and Strateos). And in February, I covered a ‘smart pill,’ developed by Tim Lu’s group at MIT, that uses engineered cells to sense molecular biomarkers in the gastrointestinal tract. The cells then relay that information, via Bluetooth, to a nearby computer. By fusing electronics and engineered cells, in other words, new medical applications emerged.
Electronics and computer programming, then, have made experiments better and cells smarter. A new paper, published last week in Science Advances, pushes this interface further still.
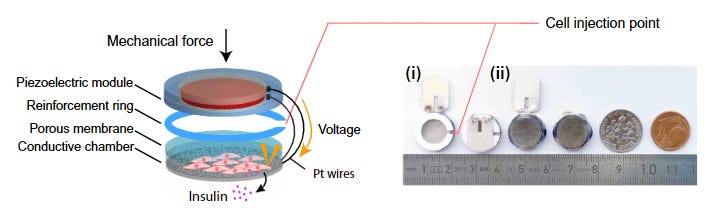
By connecting a tiny, piezoelectric membrane with ‘electro-sensing’ human cells that release insulin upon sensing a voltage, a team at ETH Zürich in Switzerland has created a miniature device that restores sugar levels in a mouse model of Type 1 Diabetes with, quite literally, the press of a button. The device has a diameter smaller than a U.S. dime.
Shocking Past
There are many ways to control genetically-engineered cells — chemicals, light, ultrasound, electricity. Each is tethered, inextricably, to a fundamental flaw.
It’s hard to steer chemicals towards a specific site in the body, for instance, which makes chemicals unfeasible for most medical applications. Light can’t penetrate very deeply into tissue or skin. Ultrasound requires somewhat expensive, specialized equipment. Electricity, then, is probably a good option; it is, after all, the bespoke language of multicellular systems.
The heart, the brain, and the nervous system all work via rivers of electrons.
Perhaps that’s why, in 2020, Fussenegger’s team engineered proinsulin-producing human cells — specifically, pancreatic beta cells called 1.1E7 — to sense external voltages and produce and release proinsulin in response. Those cells, originally described in Science, were the first cell-based therapy controlled with an ‘electro-genetics’ system. The voltages in that paper, though, were generated by an external power source.
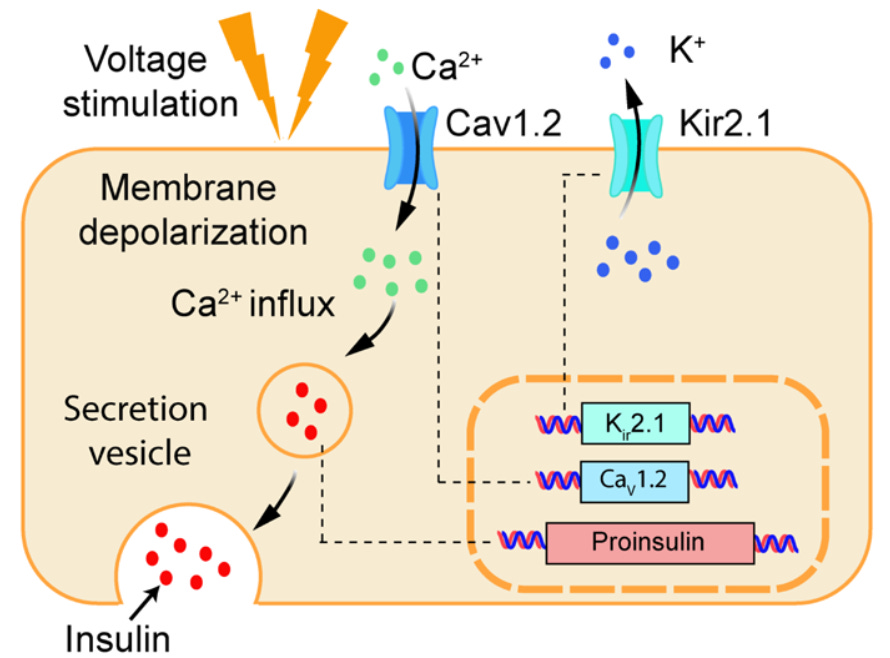
Now, two years later, Fussenegger’s team has removed the external power source and has miniaturized the electronics. All the components are now packaged into a tiny device. At one end is a piezoelectric material, made from polyvinylidene fluoride, that generates a voltage when pressed. This voltage then travels through the device and switches on the electro-sensing cells, ordering them to release insulin. The device is entirely self-powered; no batteries needed.
Into the Living
Before implanting a device into living animals, two things are needed:
A mouse model that has some condition, or phenotype, to be treated.
A device that works as expected.
Creating the mouse model, in this case, was straightforward. The researchers raised male mice to eight weeks of age, and then injected them daily, for five consecutive days, with a chemical called streptozotocin. This chemical, an antibiotic, kills pancreatic beta cells and thus reduces the amount of insulin that circulates through the bloodstream.
To test the device, electro-sensing cells were packaged into a conductive chamber within the miniature device. The button was pressed once per second, which deformed the piezoelectric membrane, which produced a voltage, which ordered the electro-sensing cells to release insulin. After three minutes of button pressing, the electro-sensing cells produced about 4 micrograms of insulin per liter of volume.
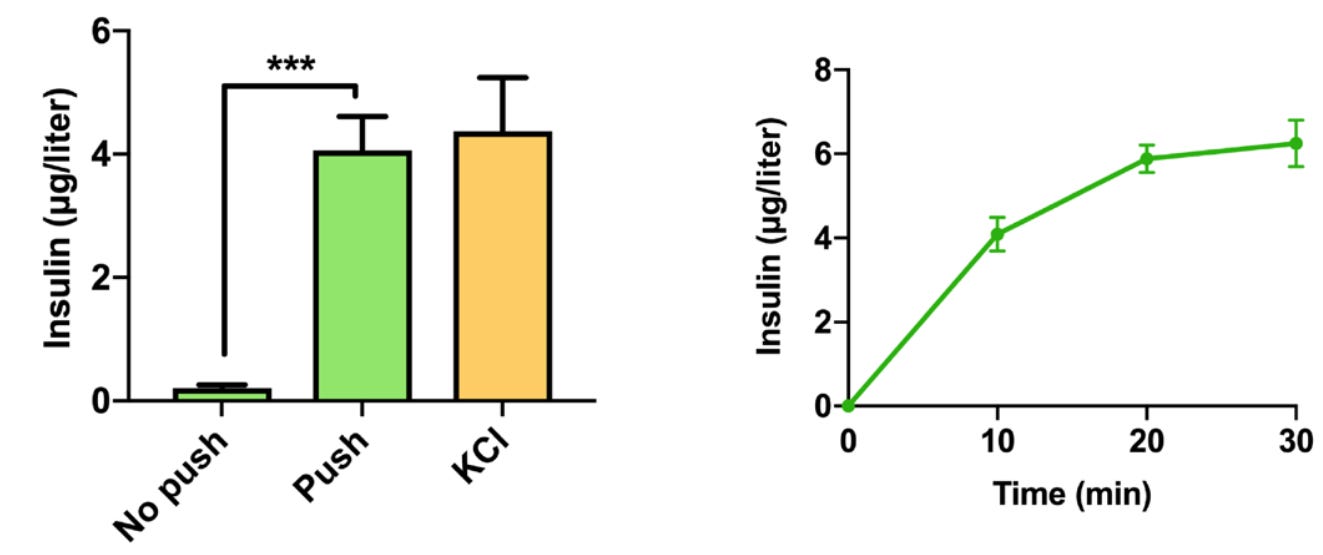
The devices were next implanted into the backsides of each mouse and closely monitored. After 30 days, a handful of mice were killed and their tissues were extracted. Implanted devices did not cause any systemic toxicity, damage to the kidneys or liver, or immune cell infiltration at the surgical site. And, after a month, each device still worked as expected — a button press still produced about 1 volt, without any noticeable drop-off in performance.
In a final experiment, the devices were implanted into mice with low insulin levels and heightened blood sugar levels (a proxy for type 1 diabetes). Animals fasted for four hours, and then each button was pressed, continuously, at a rate of one time per second. Glucose and insulin levels were measured over a four-hour period. Mice with the button-like device had a significant drop in glucose levels, and a significant elevation in insulin levels, relative to non-stimulated mice with the same device.
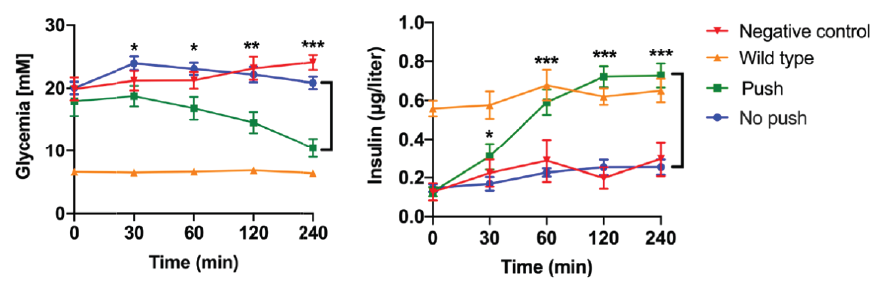
Caveats
I love this study because it’s original and useful. This device is a brilliant blend of electrical engineering and synthetic biology. I suspect it will inspire new ways of thinking at the intersection of these two disciplines.
It’s certainly too early for these devices to be useful in a clinical context. They haven’t been tested for longer than a month and any applications in people, surely, would require that these devices work for a year or more. Other devices, crafted with the same materials, have been implanted in people and validated for up to 6 months. There’s no guarantee, however, that the electro-sensing cell circuits wouldn’t mutate or break over time.
The big breakthrough in this study, I’d argue, is that this device is entirely self-powered. There are no external electronics or batteries. The device is simple and minimal; the most likely breaking point, it seems, is the cells themselves. To ensure that the engineered cells work for a year or more, perhaps one could introduce genetic redundancies (e.g. introduce backup genes for the ion transport proteins) or devise some clever means to resist mutations.
Thanks for reading.
Until next time,
— Niko // @NikoMcCarty
Email: niko@newscience.org